Real-time monitoring of 2-amino-2-methylpropan-1-ol and piperazine emissions to air from TCM post combustion CO2 capture plant during treatment of RFCC flue gas
Audun Drageseta*, Øyvind Ullestada,b, Eirik Romslo Kleppea,b, Blair McMastera,c, Magnus Aronssona,b, Jonas-Andre Olsena,b
aTechnology Centre Mongstad (TCM), 5954 Mongstad, NorwabEquinor ASA, PO Box 8500, 4035 Stavanger, Norway
cTotalEnergies EP Norge AS, Finnestadveien 44, Dusavik, 4029 Stavanger, Norway
Abstract
Monitoring and understanding the emissions of pollutants is vital for safe implementation of new industries. To ensure the safe adoption of amine-based post-combustion carbon capture to combat climate change, reliable and accurate monitoring technologies must be available for commercial projects to ensure they can monitor and control any new pollutants that might results from capturing CO2 from an industrial flue gas. As a test site for carbon capture technologies, Technology Centre Mongstad (TCM) monitors pollutants emitted in the flue gas via online sampling and analysis as per regulatory requirements. This work presents the first results from a newly installed ion-molecule reaction mass spectrometer (IMR-MS) that was employed during a test campaign with the amine solvent blend of 2-amino-2-methylpropan-1-ol (AMP) and piperazine (PZ) to monitor trace pollutants in the emitted flue gas. The primary pollutants were monitored and reported in real time in the range from 100 ppb (parts per billion) to 10 ppm (parts per million) and compared with extractive isokinetic sampling during a test campaign with an oil refinery cracker gas. The instrument allowed for real-time trending of the amine pollutants AMP and PZ in ppb range, which is the expected range required by regulators for some full-scale plants.
1. Introduction
Decarbonizing heavy industries is key for achieving the carbon mitigation goals outlined in the IPCC-6 report [1]. Amine-based carbon capture is among the most mature technologies for decarbonizing existing industrial point sources for CO2 emissions. Technology Centre Mongstad (TCM) have operated and demonstrated both non-proprietary and proprietary amine solvents for post-combustion carbon capture (PCCC) since 2012 [2]. TCM is located on the west coast of Norway in the vicinity of Equinor’s oil refinery at Mongstad. With access to two distinctly different industrial flue gases: combined-cycle gas turbine (CCGT)-based combined-heat-and-power plant (CHP) and RFCC (Residual fluid catalytic cracker) and the ability to manipulate these flue gases through air dilution and recycling of CO2, TCM can assess solvent technologies under conditions that are representative of multiple industries emissions [3]. Among the main objectives of TCMs test campaigns is to reduce the risk (economic and environmental) for commercial application and full-scale deployment of Carbon Capture and Storage (CCS). Key among the test campaigns conducted
* Corresponding author. Tel.: +47 9592 5273, E-mail address: Audun.drageset@tcmda.com
at TCM are the open test campaigns with non-proprietary solvents like aqueous 2-aminoethan-1-ol (commonly known as Monoethanolamine or MEA) and the aqueous blend of 2-amino-2-methylpropan-1-ol (AMP) and Piperazine (PZ), also known as CESAR1. Data and learnings from these campaigns can be disseminated in line with TCMs purpose to ensure safe technology adoption to combat climate change.
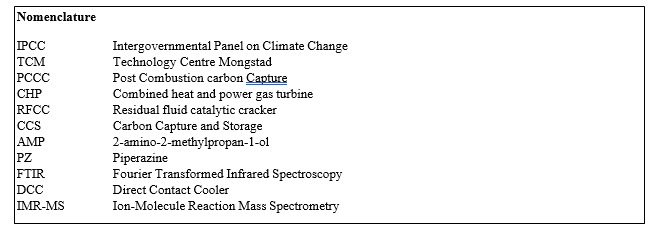
The test activities at TCM must be conducted within the framework of an emission permit [4]. The permit regulates aspects of the plant operation, among them emission to air and sets restrictions on components and their concentration. During the CO2 capture process trace amounts of contaminants are introduced to the treated gas emitted to air. Common pollutants are amines and volatile degradation products from the process like aldehydes, ketones and ammonia. Of particular concern is the class of compounds known as Nitramines and Nitrosamines, as these are known mutagenic agents and can cause cancer with prolonged exposure. In addition to direct process emissions, amines generate these compounds through photochemical reactions in the atmosphere [5]. Thus, the combination of stack emission monitoring and dispersion modelling is used to ensure the load to local environment (air and water) are within acceptable safety margins to safeguard public health and avoid damaging local ecosystems. TCM is required by the regulators to conduct continuous real-time monitoring of emissions and similar requirements may be imposed on full- scale plants in Norway and the rest of Europe. Industrial emission monitoring instruments and methods will therefore be important for full scale adoption of amine-based carbon capture. Fourier transformed infrared spectroscopy (FTIR) has been the primary instrument for real-time reporting of emissions for plant operation at TCM, but the quantification limit has been in the range of 0.5-1 ppm [2c]. Instruments with lower limits are required as the emission limit for some plants can be 1 ppm or lower [6]. Proton Transfer Reaction Time of Flight Mass spectrometry (PTR-TOF-MS) have been demonstrated in monitoring applications at TCM down to single digit ppb levels [7], however at time of writing the instrument results are not available in real-time for plant operation as the raw results recorded by the instrument require post-processing by skilled personnel. Herein we present results from a new Ion-Molecule Reaction Mass spectrometer (IMR-MS) tested during the 2020 campaign with an open solvent mixture of AMP and PZ.
2. Experimental section
2.1 Amine plant and operational conditions
TCM operates a generic amine plant built and designed by Aker Solutions and Kværner with a flue gas capacity up to 60 000 Sm3/h and which has previously been described in the literature, hence only a description of the plant configuration is disclosed below [2]. The data presented in this work is from a test period of optimized plant configuration with the RFCC flue gas (14 vol% CO2) and a solvent mixture of AMP (29 wt%) and PZ (11 wt%). The plant was operated with 18-meter packing height and a flue gas flow rate of 35 000 Sm3. The plant was optimized to reduce aerosol emissions by (1) removal of acidic sulfuric aerosols from the flue gas via pH regulation of the Direct Contact Cooler (DCC) (NaOH injection to keep pH 8) [8], (2) Brownian diffusion filter [3b] to further reduce particle count and (3) a temperature profile adjusted to promote aerosol growth and capture in the first two water washes. The plant configurations and process conditions are summarized in Table and Figure 1, additional information and overall test objectives of the campaign are available in the literature [9].
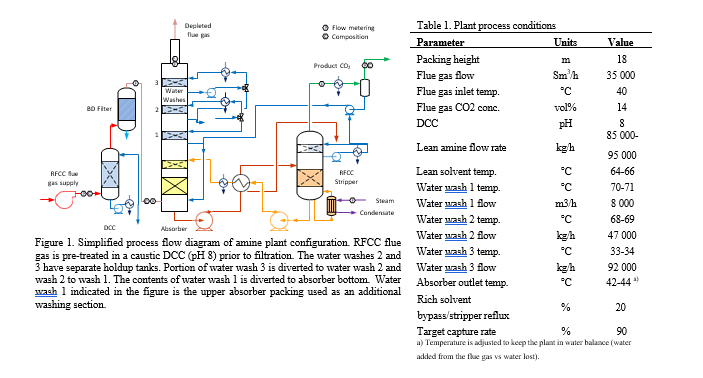
2.2 Absorber stack emission sampling
The RFCC flue gas contain acidic aerosols. Such aerosols will promote amine emissions to air, as amines are dissolved in the aerosol droplets as they travel through the absorber [10]. To acquire representative samples from such an emission point one must account for the aerosol momentum [11]. Key considerations are: (1) selection of the sample probe shape and placement, (2) the sampling rate and conditioning of the sample and (3) its transport. As a test site TCM have additional needs with respect to gas sampling and analysis for R&D applications and have therefore 3 separate sample points with comparable probes and location (Gooseneck, Paul Gothe, Heated combination probe, Version B, S-Pitot tube) (see Figure 2). Isokinetic extractive samples are collected via a cold sample system (see section 2.4) while online systems use a heated sample system (see Figure 3). The heated gas sampling system at TCM is designed to reduce measurement bias when the gas contains aerosols. The sample gas is collected via a gooseneck sample probe (SP2) heated to 120 °C. In addition, a fast-loop system is configured to sample the stack at 1 m3/h, resulting in a sampling velocity 10-11 m/s that mimics isokinetic sampling conditions. By mimicking isokinetic sampling conditions one aim to get a representative sample of the aerosols in the gas. The fast loop is equipped with a vaporizer (Elmess thermo systems, Electrical flow heater, 35 – 200 °C with modified temperature control) where the sample is heated to elevated temperature (120 °C), disrupting and evaporating the aerosols to the gaseous phase. An inline heated filter (3-micron pore size) removes solid particulates from the gas stream. A sample stream of the fast-loop sample gas is transported through an approximately 100-meter sample line (O’Brien, TRACEPAC®, TrueTube EPS, Electropolished SilcoNet tubing, 1/4 inch) to an environmentally controlled analyser-house at ground level for easy access to the analytical instruments. The final heated samples system (SP3) is connected to an analyser
house at the top of the absorber via a 10-meter heated sample line and is predominantly used for research applications [7].
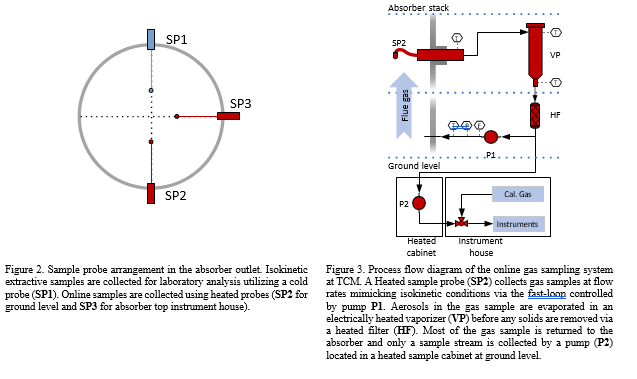
2.3 Mass spectrometry system
This work utilizes a commercially available Ion-Molecule Reaction Mass spectrometer (IMR-MS) (AirSense, V&F Analyse- und Messetchnik GmbH, Austria) [12]. Soft chemical ionisation of the sample gas is favourable to respectively reduce and minimise fragmentation of the parent molecules in a complex mixture like a flue gas containing trace volatile organic compounds. To achieve this the instrument utilises different gasses with varying ionisation energy to ionise the sample, in this work Hg (10.44 eV) and Xe (12.13 eV) ionisation modes were used. This can allow for the separation of ions with similar mass if their ionisation potential is different. The IMR-MS was installed in the ground level analyser house with the sampling system described in Figure 3. The instrument was calibrated using aqueous solutions and a calibration gas generator (HOVACAL, Digital 312-MF, IAS GmbH, Germany) with nitrogen as a carrier gas (Table 2.). The mass spectra of each component were recorded and used in selection mass signal for monitoring (see Figure 4). AMP exhibited water cross sensitivity in addition to mass fragment overlap with PZ, these effects were minimized after the implementation of enhancements (online calculations to correct for cross interaction between components and non-linear behaviour) in the instrument software suite (V&F Viewer Software, version 2.4). An overview of compound selection and enhancements are found in Table 2.
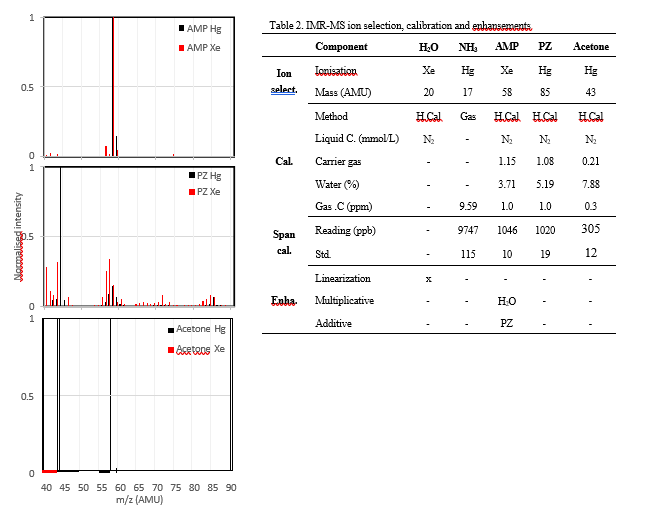
2.4 Extractive gas sampling
The extractive sampling equipment, control unit (Paul Gothe GmbH) and sample train was assembled at the sample location by TCM laboratory personnel. The probe and sample lines were at ambient temperature with a sample train consisting of three capture modes: (1) Condensation flask which cools down the gas to collect condensable, (2) Mist trap consisting of an empty impinger flask containing a 1 mm jet generating approximate gas speed of 80 m/s that that collects medium/large aerosols and (3) absorption flasks containing sulfuric acid (0.05M) and a 1mm jet captures small aerosols, gaseous amines and ammonia in the acidic solution (see Figure 5). Generally sampling time is 1-2 hours. Samples are analysed using a Dionex Integrion HPIC System (model ICS-5000, Thermo Fisher Scientific) which included an IonPac CS19 column and an IonPac CG19 guard column and MS detector (Thermo Fisher, ISQ EC Mass spectrometer). Aldehydes and acetone are sampled using a similar sample train where the absorption bottles are exchanged with two silica cartridges (Sep-Pak® DNPH-Silica Cartridge, 800 mg). Sample time is considerably shorter as cartridges have lower capacity than absorption flasks, all cartridges were analysed by a third-party lab. Isokinetic gas sampling was performed on five occasions during the test period.
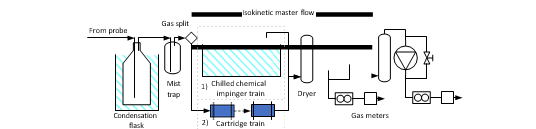
3. Results and discussion
3.1 Plant stability
During the test period minor plant upsets occurred resulting in unstable plant conditions in the following hours (see Figure 6 and Table 3). The instruments capture the impact on process emissions prior to reaching a steady state. Shorter process upsets have negligible impact and emissions are relatively stable. However more complex situations or extended downtime can result in a significant elevation of emissions during a limited period. Accommodating for increased emissions during such eventualities should be described in the plant emission permit to ensure regulators and operators are aligned on acceptable limits that give operators the required flexibility to stabilise the plant in such scenarios.
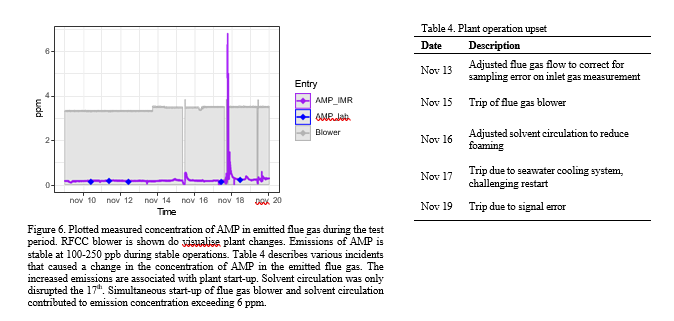
3.2 Result evaluation
The incidents described above do not represent stable process conditions and are therefore removed from the dataset when comparing the performance of the IMR-MS instrument to established extractive sampling methods. Figure 7 shows violin plots of the cleaned online dataset and extractive lab sampling. Violin plots are similar to box plots but also visualize the distribution of measurements. Among the extractive samples, AMP results have the best agreement with the extractive samples (Figure 7a). As AMP was shown to be the primary emission component in previously
reported work [11], care was taken to implement enhancements to mitigate cross-sensitivity affecting AMP during calibration. The water content in the flue gas was the main component impacting the AMP signal, this was resolved by adding a linearization to the water signal in addition to a multiplicative enhancement with AMP. As seen in Figure 4 PZ has a fragmentation pattern that overlaps with the selected mass for AMP (see Table 2). The impact of this interaction is reduced with an enhancement (Table 2), as a result AMP is monitored with high accuracy.
The calibration of PZ was challenging when using the available aqueous solutions and Hovacal setup. The component is sticky and adheres to the surfaces of the calibration equipment and lines. Therefore, achieving saturation of the Hovacal and inlet lines to the instrument were time consuming (30-60 minutes). A potential consequence is that a zero or span calibration was performed before the system had reached equilibrium, as is suggested in Figure 8, pointing to a bias in the span calibration. This could explain the instrument bias shown in Figure 7b.
Figure 7c shows poor agreement between the IMR-MS and the extractive samples for acetone. No clear explanation could be discerned by examining the calibration logs or setup. Further investigation is required.
Ammonia on the IMR-MS overestimates the concentration as can be seen in Figure 7d, however this is due to a calibration bias as is evident when comparing with FTIR results (see Figure 9). The instruments follow the same trends, and the bias should be resolved by improved the instrument calibration.
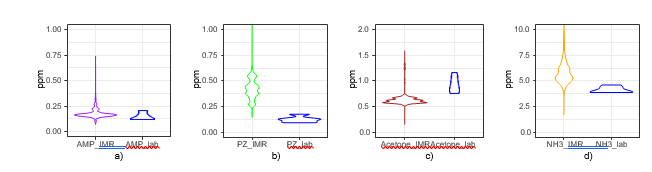
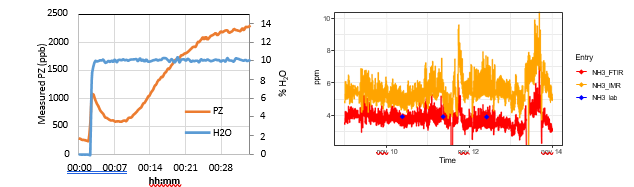
4. Conclusion
In this work we have successfully demonstrated the application of a new IMR-MS instrument for real-time monitoring and reporting of contaminants in the treated flue gas for an amine-based post-combustion carbon capture process. The instrument was installed in an industrial environment and measured the main emission components regulated in the emission permit of TCM.
The real-time monitoring and reporting of 2-methyl-2-aminopropan-1-ol (AMP) emissions was successfully conducted with a new IMR-MS instrument at TCM. The instrument accurately reported AMP in a range of 100-250 ppb. The instrument also reported PZ, acetone and ammonia but with lower accuracy. This was predominantly due to sub optimal calibration setups and procedures resulting in a systematic error. To mitigate some of the challenges experienced in this work dry calibration gasses should be used, preferably certified gas bottles. In addition, a more thorough screening of the flue gas contaminants and potential cross interactions of all contaminants should be assessed. This work demonstrates that commercial instruments capable of monitoring emissions in ppb range are available on the market. With application tailored calibration the instrument can be adapted to different flue gas sources and
capture solvents.
5. Acknowledgment
The authors gratefully acknowledge the staff of TCM DA, Gassnova, Equinor, Shell and TotalEnergies for their contribution and work at the TCM DA facility. The authors also gratefully acknowledge Gassnova, Equinor, Shell, and TotalEnergies as the owners of TCM DA for their financial support and contributions.
References
- IPCC, 2021: Summary for Policymakers. In: Climate Change 2021: The Physical Science Basis. Contribution of Working Group I to the Sixth Assessment Report of the Intergovernmental Panel on Climate Change [MassonDelmotte, Zhai VP, Pirani A, Connors SL, Péan C, Berger S, Caud N, Chen Y, Goldfarb L, Gomis MI, Huang M, Leitzell K, Lonnoy E, Matthews JBR, Maycock TK, Waterfield T, Yelekçi O, Yu R, and Zhou B (eds.)]. Cambridge University Press.
- a) Gjernes E, Pedersen S, Jain D, Åsen KI, Hvidsten OA, de Koeijer G, Faramarzi L, de Cazenove T, Documenting modes of operation with cost saving potential at the Technology Centre Mongstad, 14th Greenhouse Gas Control Technologies Conference Melbourne 21-26 October 2018 (GHGT-14), b) Faramarzi L, Thimsen D , Hume S, Maxon A, Watson G, Pedersen S , Gjernes E, Fostås BF, Lombardo G, Cents T, Morken AK, Shah MI, de Cazenove T, Hamborg ES, Energy Procedia, 114, 2017, 1128-1145, c) Morken A, Pedersen S, Kleppe ER, Wisthaler A, Vernstad K , Ullestad Ø , Flø NE, Faramarzi L, Hamborg ES, Energy Procedia, 114, 2017, 1245-1262, d) Benquet C, Knarvik A, Gjernes E, Hvidsten OA, Kleppe ER, Akhter S, First Process Results and Operational Experience with CESAR1 Solvent at TCM with High Capture Rates (ALIGN-CCUS Project), Proceedings of the 15th Greenhouse Gas Control Technologies Conference 15-18 March 2021.
- a) Johnsen K, Kleppe ER, Faramarzi L, Benquet C, Gjernes E, de Cazenove T , Morken AK, Flø N, Shah MI, Aronsson M, Ullestad Ø, CO2 product quality: assessment of the range and level of impurities in the CO2 product stream from MEA testing at the Technology Centre Mongstad (TCM), Proceedings of the 14th Greenhouse Gas Control Technologies Conference Melbourne 21-26 October 2018 (GHGT-14), b) Lombardo G, Shah MI, Fostås B, Hvidsten OA, Faramarzi L, de Cazenove T, Lepaumier H, Rogiers P, Results from testing of a Brownian diffusion filter for reducing the aerosol concentration in a residual fluidized catalytic cracker flue gas at the Technology Centre Mongstad, 14th Greenhouse Gas Control Technologies Conference Melbourne 21-26 October 2018 (GHGT-14).
- TCM emission permit, Permit Nr.: 2011.0257T, Plant Nr.: 1263.0105.01 – accessed 02.08.2022. https://www.norskeutslipp.no/WebHandlers/PDFDocumentHandler.ashx?documentID=577603&documentType=T&companyID=25735&aar=0&epslanguage=no
- a) Nielsen CJ, Herrmann H, Weller C, Chem. Soc. Rev., 41, 2012, 6684-6704, b) Låg M, Andreassen Å, Instanes C, Lindeman B, Health effects of amines and derivatives associated with CO2 capture, Norwegian Institute of Public Health, 2011, c) Gjernes E, Helgensen LI, Maree Y, Energy Procedia, 37, 2013, 735-742, d) de Koeijer G, Talstad VR, Nepstad S, Tønnesen D, Falk-Pedersen O, Maree Y, Nielsen CJ, 2013, 18, 200-207, e) Tan W, Zhu L, Mikoviny T, Nielsen CJ, Wisthaler A, D’Anna B, Antonsen S, Stenstrøm Y, Farren NJ, Hamilton JF, Boustead GA, Brennan AD, Ingham T, Heard DE. J. Phys. Chem. A, 125, 2021, 411-422, f) Tan W, Zhu L, Mikoviny T, Nielsen CJ, Tang Y, Wisthaler A, Eichler P, Müller M, D’Anna B, Farren NJ, Hamilton JF, Petterson JBC, Halliquist M, Antonsen S, Stenstrøm Y, J. Phys. Chem. A, 125, 2021, 7502-7519.
- Tønnesen D, CO2-capture at Klemetsrud. Modelling of nitros- and nitramines, Norwegian Institute for Air Research, NILU report 11/2018. https://hss.miljodirektoratet.no/api/1/publisert/hoering/vedlegg/16832, accessed 20.07.20
- a) Languille B, Drageset A, Mikoviny T, Zardin E, Benquet C, Ullestad Ø, Aronson M, Kleppe ER, Wisthaler A, Best practices for the measurement of 2-amino-2-methyl-1-propanol, piperazine and their degradation products in amine plant emissions, 15th Greenhouse Gas Control Technologies Conference Abu Dhabi 15-18 March 2021 (GHGT-15), b) Languille B, Drageset A, Mikoviny T, Zardin E, Benquet C, Ullestad Ø, Aronsson M, Kleppe ER, Wisthaler A, Atmospheric emissions of amino-methyl-propanol, piperazine and their degradation products during the 2019-20 ALIGN-CCUS campaign at the Technology Centre Mongstad, 15th Greenhouse Gas Control Technologies Conference Abu Dhabi 15-18 March 2021 (GHGT-15).
- Akinpelumi K, Saha C, Rochelle GT, Piperazine aerosol mitigation for post-combustion carbon capture, International Journal of Greenhouse Gas Control, 2019, 91, 102845.
- Hume, SA McMaster, Drageset A, Shah, MI, Kleppe ER, Results from CESAR1 testing at the CO2 Technology Centre Mongstad. Verification of Residual Fluid Catalytic Cracker (RFCC) baseline results, 16th Greenhouse Gas Control Technologies Conference Lausanne 23-27 October 2022 (GHGT-16).
- a) de Cazenove T, Bouma RHB, Goetheer ELV, van Os PJ, Hamborg ES, Aerosol Measurement Technique: Demonstration at CO2 Technology Centre Mongstad, Energy Procedia, 2016, 86, 160-170, b) Lombardo G, Fostås BF, Shah MI, Morken AK, Hvidsten OA, Mertens J, Hamborg ES, Results from aerosol measurement in amine plant treating gas turbine and Residue Fluidized Catalytic Cracker flue gases at the CO2 Technology Centre Mongstad, 13th Greenhouse Gas Control Technologies Conference Lausanne 14-18 November 2016 (GHGT-13).
- Lodge JrJP, Methods of Air sampling and Analysis, Third edition, CRC Press, 1989.
- a) Sauer C, Lorén A, Schefer A, Carlsson P-A, On-Line Composition Analysis of Complex Hydrocarbon Streams by Time-Resolved Fourier Transform Infrared Spectroscopy and Ion−Molecule Reaction Mass Spectrometry, Analytical Chemistry 2021, 93, 13187-13195, b) Wang Y, Han H, Shen S, Li J, Wang H, Chu Y, Control of solvent use in medical devices by proton transfer reaction mass spectrometry and ion molecule reaction mass spectrometry, Journal of Pharmaceutical and Biomedical Analysis, 2009, 50, 252-256, c) Dearth MA, Evaluation of a Commercial Mass Spectrometer for Its Potential To Measure Auto Exhaust Constituents in Real Time, Industrial & Engineering Chemistry Research, 1999, 38, 2203-2209.
Atmospheric emissions of amino- methyl-propanol, piperazine and their degradation products during the 2019–20 ALIGN-CCUS campaign at the Technology Centre Mongstad (2021)
Baptiste Languillea, Audun Dragesetb, Tomas Mikovinya, Erika Zardina, Christophe Benquetb,c, Øyvind Ullestadb,d, Magnus Aronsonb,d, Eirik Romslo Kleppeb,d, Armin Wisthalera*
aUniversity of Oslo, Department of Chemistry, P.O. Box 1033 Blindern, 0351 Oslo, Norway bTechnology Centre Mongstad (TCM), 5954 Mongstad, Norway cTotal E&P Norge, Finnestadveien 44, Dusavik, 4029 Stavanger, Norway dEquinor ASA, PO Box 8500, 4035 Stavanger, Norway *Corresponding author
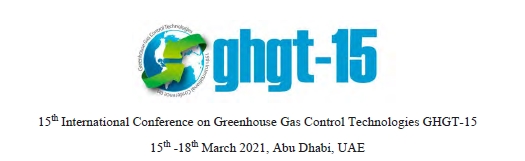
Abstract
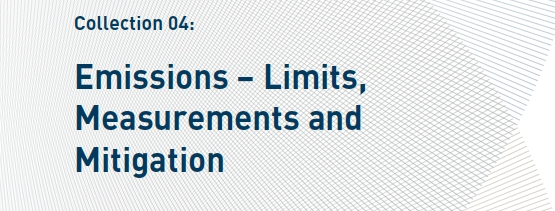
In the frame of the 2019-20 ALIGN-CCUS campaign, the amine plant at the Technology Centre Mongstad (TCM) was operated with the CESAR 1 solvent, i.e. an aqueous solution of 2-amino-2-methylpropan-1-ol (AMP) and piperazine (PZ), for removing carbon dioxide from the flue gas of Equinor’s combined cycle gas turbine plant. An online Proton-Transfer-Reaction Time-of- Flight Mass Spectrometer (PTR-TOF-MS) was used for quantifying atmospheric emissions of AMP and PZ, as well as emissions of amine degradation products and solvent impurities. Mean and median AMP levels emitted to the atmosphere over an operational period of 13 weeks were 562 and 377 ppb, respectively. PZ emissions to the atmosphere were much lower, with mean and median levels being 6.0 and 0.4 ppb, respectively. Three small carbonyl species (formaldehyde, acetaldehyde, acetone) were emitted at levels of tens to hundreds of ppb. Nitrogen-containing degradation products and impurities of solvent amines with mean emission levels >1 ppb included the following compounds: monomethylamine (MMA), formamide (FA), morpholine (MOR), 4,4- dimethyloxazolidine (DMO), 2-methyl-2-(methylamino)propan-1-ol (MeAMP), 4-acetylmorpholine (AMOR) and a compound with a molecular sum formula of C8H14N2, which we tentatively assigned to an alkylated imidazole or pyrazole. Low (<5 ppm) emissions of AMP were associated with a low flue gas temperature (<38 °C) and large temperature gradient between the two water wash sections.
1. Introduction
Amine-based post-combustion carbon capture (PCCC) is the technologically most mature solution for removing carbon dioxide (CO2) from a flue gas stream. The reduction in CO2 emissions comes, however, at the expense of amine emissions to the atmosphere. Atmospheric oxidation processes may partially transform the emitted amines into nitrosamines and nitramines [1], which are substances with known carcinogenic or potential carcinogenic properties. Since it is not possible to monitor and surveil nitrosamines and nitramines at ambient sub-ng/m³ levels, exposure to nitrosamines and nitramines is usually calculated taking into account dispersion and atmospheric processing of emitted amines. A key input parameter to such calculations is the emission rate of solvent amines and of smaller amines formed during solvent degradation. Amine emission data are thus of pivotal importance for ensuring an environmentally safe operation of an amine-based PCCC plant. A recent review by Scottish Environment Protection Agency [2] concludes that there is limited open source data available on amine emissions to the atmosphere and that most available data is based on capture solvents such as monoethanolamine (MEA) that are not necessarily representative of the mix of solvents used in state-of-the-art PCCC plants.
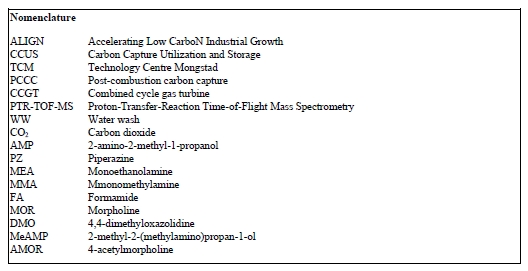
The Technology Centre Mongstad (TCM) (www.tcmda.com) in Norway is one of the world’s leading facilities for testing and improving CO2 capture technologies. TCM’s tasks also include the assessment of amine emissions to the atmosphere and the development of emission reduction strategies. Only few results from the emission measurements have, however, hitherto been disclosed. This is because detailed emission data would reveal the chemical composition of the solvent, which is, in most cases, confidential business information. Therefore, only MEA emission data are currently available in the open literature [3, 4].
ALIGN-CCUS (Accelerating Low CarboN Industrial Growth through Carbon Capture Utilisation and Storage) is a project financed through the first ERA-NET Co-fund ACT program funded by nine European countries and the European Union Horizon 2020 program. The ALIGN consortium includes 31 partners from industry, research and academia and has considerable involvement of industrial companies and an enterprise organization. The ALIGN- CCUS project aims at accelerating the transition of current industry and power sectors into a future of continued economic activity and low-carbon emissions, in which carbon capture, utilization and storage (CCUS) plays an essential role. For optimizing and reducing costs of PCCC, the ALIGN consortium has implemented test programs at four different pilot plants and testing facilities including TCM, the SINTEF pilot rig at Tiller/Trondheim in Norway, RWE’s Coal Innovation Centre at Niederaussem in Germany, and the Pilot-scale Advanced CO2 Capture Technology (PACT) facilities in Sheffield in the United Kingdom. TCM’s contribution to the ALIGN-CCUS project is aimed at bridging knowledge gaps as well as reducing HSE, technical and financial risks of technology upscaling.
In the 2019-20 ALIGN-CCUS campaign at TCM, flue gas from Equinor’s combined cycle gas turbine (CCGT) plant in Mongstad was treated with the aqueous 2-amino-2-methylpropan-1-ol (AMP) / piperazine (PZ) (CESAR 1) solvent. The CESAR 1 solvent was selected due to its lower energy consumption and higher stability as compared to MEA. Among the topics of the study were emission control and monitoring as well as solvent consumption. The main goal of the campaign was to demonstrate that this advanced amine solvent can be used at a large scale and with a real flue gas.
Results from the ALIGN-CCUS project are in the open domain, and we are herein reporting, for the first time, atmospheric emission data of AMP, PZ and their degradation products.
2. Method
The ALIGN-CCUS 2019-2020 campaign was carried out at TCM from September 12, 2019 to January 10, 2020. Flue gas from Equinor’s CCGT plant in Mongstad was treated in TCM’s amine plant operating with the CESAR 1 solvent. The chemical-analytical set-up to measure atmospheric emissions of amines, amine impurities and amine degradation products is described in detail in a companion paper [5]. Herein, we only use the data collected with an online Proton-Transfer-Reaction Time-of-Flight Mass Spectrometer (PTR-TOF-MS) [3]. The calibration of AMP and PZ is also described in the companion paper. Formaldehyde, acetaldehyde and acetone as well as acetonitrile were calibrated using a dynamically diluted calibration gas standard (Apel-Riemer Environmental, Inc., Miami, FL, USA). All other compounds are reported as acetone-equivalents, i.e. the acetone response factor was used for signal quantification. Reported volume mixing ratios have an accuracy of ±10% for calibrated compounds, and ±50% for compounds reported as acetone-equivalents.
3. Results and discussion
3.1 Atmospheric emissions of AMP and PZ
Figure 1 shows the distribution of AMP and PZ volume mixing ratios, respectively, as measured during the 2019- 2020 ALIGN-CCUS campaign at TCM. Mean and median AMP levels emitted were 562 and 377 ppb, respectively. PZ emissions were much lower, with mean and median levels being 6.0 and 0.4 ppb, respectively. The mean value is significantly higher than the median value because of sporadic PZ bursts. No experimental data are available in the open literature to compare our data with. Kolderup et al. [6] carried out modeling simulations and estimated total AMP and PZ emissions in the 0.55 – 30 ppm range. The AMP levels observed in this study were similar to MEA levels found in previous work at TCM [7, 4, 8]. Notably, AMP emissions were typically three orders of magnitude higher than PZ emissions. Khakharia et al. [9] reported an AMP-to-PZ emission ratio of 26, while Mertens et al. [10] found two orders of magnitude higher AMP emissions as compared to PZ. This discrepancy cannot be explained by the AMP-to-PZ-ratio in the solvents (3 M AMP and 1.8 M PZ in the Mertens et al. study [10]; 3.6 M AMP and 0.9 M PZ in the Khakharia et al. study [9]; 3 M kg-1 AMP and 1.5 M kg-1 PZ in the CESAR 1 solvent). The low PZ emission levels observed during the ALIGN-CCUS campaign can be explained by a low entrainment rate of PZ into the gas phase and/or more efficient scrubbing of PZ in the water wash sections.
3.2 Atmospheric emissions of amine degradation products and impurities
10 amine degradation products and 1 solvent impurity were emitted to the atmosphere with a mean and median volume mixing ratio above 1 ppb during the ALIGN-CCUS 2019-2020 campaign (Figure 2). Three small carbonyl species (formaldehyde, acetaldehyde, acetone) were emitted at levels of tens to hundreds of ppb, with acetone reaching the highest levels. It was observed previously [12, 13] that acetone is a major decomposition product of an aqueous AMP/PZ blend. Seven of the remaining species that were emitted to the atmosphere included one nitrogen atom: monomethylamine (MMA), formamide (FA), morpholine (MOR), 4,4-dimethyloxazolidine (DMO), 2-methyl-2- (methylamino)propan-1-ol (MeAMP), and 4-acetylmorpholine (AMOR). The remaining compound has a molecular sum formula of C8H14N2, which we tentatively assign to an alkylated imidazole or pyrazole. Notably, MMA was emitted at a mean level of almost 20 ppb. Wang [12] expected MMA to be formed from AMP degradation but did not detect it. Wang [12] also observed the formation of formamide when exposing AMP to UV radiation. MeAMP is a common impurity of AMP and DMO has been observed as a decomposition compound in the liquid phase [12]. But so far, they have not been reported as being released to the atmosphere. MOR, AMOR and C8H14N2 have not been reported previously. It is also noteworthy that 1-nitrosopiperazine was detected in the emitted flue gas. A tentative quantification puts emission levels well below 1 ppb, but more calibration work is needed for quantifying nitrosamines.
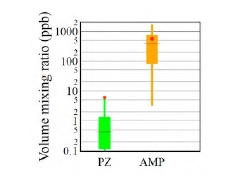
Fig. 1. Distribution of volume mixing ratios of PZ and AMP emitted to the atmosphere when flue gas from Equinor’s CCGT plant in Mongstad was scrubbed of CO2 in TCM’s amine plant operating with the CESAR 1 solvent. Boxes represent 25th and 75th percentiles, the horizontal line is the median, the dot is the mean, and whiskers represent 5th and 95th percentiles.
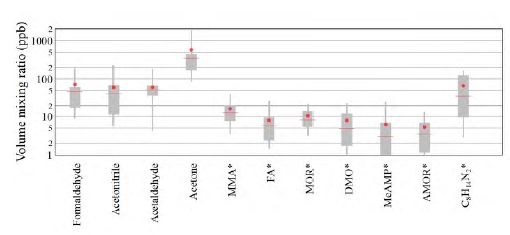
Fig. 2. Distribution of the 10 amine degradation products and 1 solvent impurity (MeAMP) that were emitted to the atmosphere with a mean level. > 1 ppb. Boxes represent 25th and 75th percentiles, the horizontal line is the median, the dot is the mean, and whiskers represent 5th and 95th percentiles. Compounds marked with an asterisk (*) are reported as in acetone-equivalent mixing ratios (see text).
3.3 Impact of the operational parameters of the plant
The operating parameters of the amine plant obviously influence the level of emissions. Mertens et al. [10] found that emissions depend upon the flue gas temperature and the temperature gradient over the water wash (WW) section. Our initial analysis thus focused on the effect of these two parameters on atmospheric emissions. Figure 3 (upper panel) shows that high (>5 ppm) AMP emissions were associated with high (>38 °C) flue gas temperatures. Figure 3 (lower panel) on the other hand shows that high AMP emissions only occurred when the temperature gradient between the two WW sections was low. Emissions of PZ and degradation products exhibited similar trends. More details will be disclosed in a forthcoming paper.
Our findings are thus in agreement with the observations by Mertens et al. [10], and this information may be used to mitigate emissions from amine plants. Previous work [9, 11] has, however, also shown that other parameters such as solvent temperature, pH and CO2 content significantly impact atmospheric emissions. The effect of additional parameters will be investigated in future work.
Finally, we would like to point out that the results shown here were obtained when the amine plant was operated with flue gas from the CCGT plant. This flue gas has a low acidic content, which prevents the formation of aerosol particles (“mist”) in the absorber column. Currently ongoing studies indicate that emission profile changes significantly when the amine plant runs on the other flue gas available at TCM, which is from the Residue Fluid Catalytic Cracker (RFCC). The results from these ongoing studies will be presented in future work.
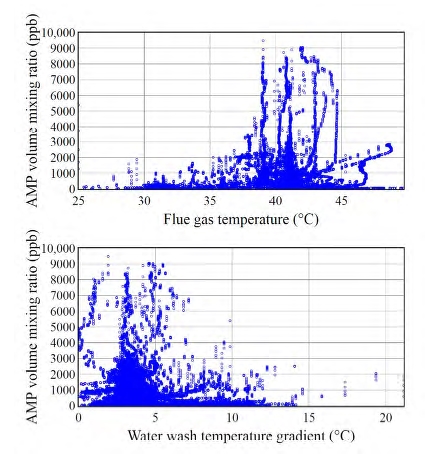
Fig. 3. Scatter plots of AMP emissions versus flue gas temperature (upper panel) and AMP emissions versus the temperature gradient over the water wash sections (lower panel). Data associated with flue gas temperatures below 25 °C were not included in the analysis. Such low temperatures only occur during the plant warm-up and not during regular plant operation.
Conclusions
We herein report, for the first time, the levels of AMP and PZ as well as of their degradation products that are emitted into the atmosphere from a PCCC plant, which was operated on the CESAR 1 solvent. Significant emissions of AMP and of two small reactive carbonyls (formaldehyde, acetaldehyde) will need to be considered in any atmospheric impact assessment study, while minor emissions of other degradation products (in particular of 1- nitrosopiperazine) warrant further investigations. We also characterized the impact of specific plant parameters on emissions and found that high (>5 ppm) AMP emissions were associated with a high flue gas temperature (>38 °C) and small temperature gradient between the two water wash sections. A more in-depth analysis of the collected data will allow us to give recommendations for mitigating emissions from amine-based PCCC plants operating on the advanced CESAR 1 solvent.
Acknowledgements
ACT ALIGN-CCUS Project No 271501. This project has received funding from RVO (NL), FZJ/PtJ (DE), Gassnova (NO), UEFISCDI (RO), BEIS (UK) and is co-funded by the European Commission under the Horizon 2020 programme ACT, Grant Agreement No. 691712 (www.alignccus.eu).
The authors gratefully acknowledge the staff of TCM DA, Gassnova, Equinor, Shell and TotalEnergies for their contribution and work at the TCM DA facility. The authors also gratefully acknowledge Gassnova, Equinor, Shell, and Total as the owners of TCM DA for their financial support and contributions.
References
- Nielsen CJ, Herrmann H, and Weller C. Atmospheric chemistry and environmental impact of the use of amines in carbon capture and storage (CCS). Chem. Soc. Rev., 41 :6684–6704, 2012.
- SEPA Scottish Environment Protection Agency. Review of amine emissions from carbon capture systems. Stirling, UK, pages 1–86, 2015.
- Zhu L, Schade G, and Nielsen CJ. Real-time monitoring of emissions from monoethanolamine-based industrial scale carbon capture facilities. Environmental science & technology, 47, 11 2013.
- Morken A, Pedersen S, Kleppe ER, Wisthaler A, Vernstad K, Ullestad Ø, Flø NE, Faramarzi L, and Hamborg ES. Degradation and emission results of amine plant operations from MEA testing at the CO2 Technology Centre Mongstad. Energy Procedia, 114:1245–1262, 07 2017.
- Languille B, Drageset A, Mikoviny T, Zardin E, Benquet C, Ullestad Ø, Aronson M, Kleppe ER, Wisthaler A. Best practices for the measurement of 2-amino-2-methyl-1-propanol, piperazine and their degradation products in amine plant emissions, 15th International Conference on Greenhouse Gas Control Technologies, GHGT-15, 15th 18th March 2021 Abu Dhabi, UAE
- Kolderup H, Silva E, Mejdell T, Tobiesen A, Haugen G, Hoff KA, Josefsen K, Strøm T, Furuseth O, Hanssen KF, Wirsching H, Myhrvold T, and Johnsen K. Emission reducing technologies amine. SINTEF Materials and Chemistry, pages 1 – 123, 2011.
- Morken A, Nenseter B, Pedersen S, Chhaganlal M, Feste JK, Tyborgnes RB, Ullestad Ø, Ulvatn H, Zhu L, Mikoviny T, Wisthaler A, Cents A, Bade O, Knudsen J, Koeijer G, Pedersen OF, and Hamborg ES. Emission results of amine plant operations from MEA testing at the CO2 Technology Centre Mongstad. Energy Procedia, 63: 6023–6038, 12 2014.
- Zhu L, Mikoviny T, Morken AK, Tan W, and Wisthaler A. A compact and easy-to-use mass spectrometer for online monitoring of amines in the flue gas of a post-combustion carbon capture plant. International Journal of Greenhouse Gas Control, 78:349 – 353, 2018.
- Khakharia P, Brachert L, Mertens J, Anderlohr C, Huizinga A, Fernandez ES, Schallert B, Schaber K, Vlugt TJH and Goetheer E. Understanding aerosol based emissions in a post combustion CO2 capture process: Parameter testing and mechanisms. International Journal of Greenhouse Gas Control, 34:63 – 74, 2015.
- Mertens J, Knudsen J, Thielens ML, and Andersen J. On-line monitoring and controlling emissions in amine post combustion carbon capture: A field test. International Journal of Greenhouse Gas Control, 6:2–11, 01 2012.
- Fulk SM and Rochelle GT. Quantification of gas and aerosol-phase piperazine emissions by FTIR under variable bench-scale absorber conditions. Energy Procedia, 63:871 – 883, 2014. 12th International Conference on Greenhouse Gas Control Technologies, GHGT-12.
- Wang T. Degradation of Aqueous 2-Amino-2-methyl-1-propanol for Carbon Dioxide Capture. PhD thesis, 2013.
- Wang T and Jens KJ. Oxidative degradation of aqueous PZ solution and AMP/PZ blends for post-combustion carbon dioxide capture. International Journal of Greenhouse Gas Control, 24:98 – 105, 2014.
Best practices for the measurement of 2-amino-2- methyl-1-propanol, piperazine and their degradation products in amine plant emissions (2021)
Baptiste Languillea, Audun Dragesetb, Tomas Mikovinya, Erika Zardina, Christophe Benquetb, Øyvind Ullestadb, Magnus Aronsonb, Eirik Romslo Kleppeb, Armin Wisthalera*
aUniversity of Oslo, Department of Chemistry, P.O. Box 1033 Blindern, 0351 Oslo, Norway bTechnology Centre Mongstad (TCM), 5954 Mongstad, Norway cTotal E&P Norge, Finnestadveien 44, Dusavik, 4029 Stavanger, Norway dEquinor ASA, PO Box 8500, 4035 Stavanger, Norway *Corresponding author
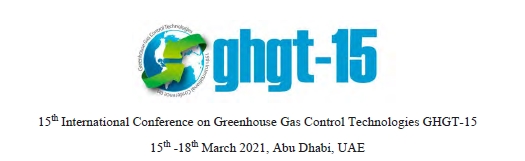
Abstract
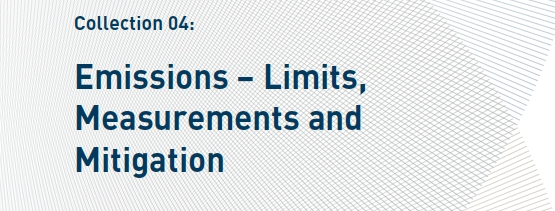
We herein present the chemical-analytical setup used to measure atmospheric emissions of amines and amine degradation products from an amine-based post-combustion carbon capture plant. The emission measurements were carried out at the Technology Centre Mongstad (TCM) in Norway, in the frame of the ALIGN-CCUS campaign from September 2019 to January 2020, when the amine plant was operated with the CESAR 1 solvent. This advanced solvent is an aqueous solution of 2-amino-2-methyl-1-propanol (AMP) and piperazine (PZ). Four chemical-analytical techniques were deployed for characterizing emission of AMP, PZ and their degradation products: online Fourier Transform Infrared (FTIR) Spectroscopy, online Proton-Transfer-Reaction Time-of-Flight Mass Spectrometry (PTR-TOF-MS), online Proton-Transfer-Reaction Quadrupole Mass Spectrometry (PTR-QMS), as well as manual impinger sampling followed by offline Ion Chromatography Mass Spectrometry (IC-MS) analysis. AMP was detected by all four methods, with the results being in reasonably good agreement. PZ was detected by PTR-TOF-MS, PTR-QMS and IC-MS, but because of the low emission levels (single-digit ppb) the latter two methods suffered from a positive bias (due to an interfering compound) and a large measurement uncertainty, respectively. 17 amine degradation products were only detected by the PTR- ToF-MS analyzer. We present exemplary results from the emission measurements carried out during the ALIGN-CCUS 2019- 2020 campaign and share some of the lessons learned from this exercise.
1. Introduction
Amine-based post-combustion carbon capture (PCCC) is the technologically most mature solution for removing carbon dioxide (CO2) from a flue gas stream. The reduction in CO2 emissions does, however, come at the expense of amine emissions to the atmosphere [1]. Atmospheric oxidation processes may partially transform the emitted amines into nitrosamines and nitramines [2], which are substances with known carcinogenic or potential carcinogenic properties. Based on a conservative risk analysis, inhalation exposure to the sum of PCCC-derived nitrosamines and nitramines should be kept below an annual average concentration of only 0.3 ng m-3 [3] for the general public. Since it is not possible to monitor such low concentrations in the atmosphere, exposure to nitrosamines and nitramines is calculated taking into account emission rates of amines, as well as the dispersion and atmospheric processing of emitted amines. Amine emission rates need to be measured, but their low concentrations (typically 0.1-10 ppm) and the high humidity levels in the treated flue gas make this a challenging analytical effort.
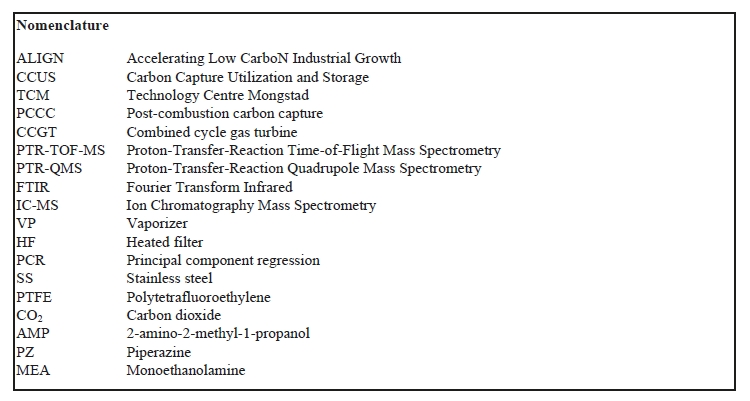
The Technology Centre Mongstad (TCM) (www.tcmda.com) in Norway is one of the world’s leading facilities for testing and improving CO2 capture technologies. TCM’s tasks also include the validation and optimization of emission sampling and measurement techniques. TCM has put considerable efforts into the characterization of atmospheric emissions and nowadays routinely monitors amines in the absorber effluent stream using a variety of analytical methods [4]. These include online Fourier Transform Infrared (FTIR) Spectroscopy, online Proton-Transfer-Reaction Time-of-Flight Mass Spectrometry (PTR-TOF-MS) [5], online Proton-Transfer-Reaction Quadrupole Mass Spectrometry (PTR-QMS) [6], as well as manual impinger sampling followed by offline Ion Chromatography Mass Spectrometry (IC-MS) analysis [4]. While amine emission data are routinely reported to the authorities, only few results have hitherto been publicly disclosed because the solvent composition is, in most cases, confidential.
ALIGN-CCUS (Accelerating Low CarboN Industrial Growth through Carbon Capture Utilisation and Storage) is a project financed through the first ERA-NET Co-fund ACT program funded by nine European countries and the European Union Horizon 2020 program. The ALIGN consortium includes 31 partners from industry, research and academia and has considerable involvement of industrial companies and an enterprise organization. The ALIGN- CCUS project aims at accelerating the transition of current industry and power sectors into a future of continued economic activity and low-carbon emissions, in which carbon capture, utilization and storage (CCUS) plays an essential role. For optimizing and reducing costs of PCCC, the ALIGN consortium has implemented test programs at four different pilot plants and testing facilities including TCM, the SINTEF pilot rig at Tiller/Trondheim in Norway, RWE’s Coal Innovation Centre at Niederaussem in Germany, and the Pilot-scale Advanced CO2 Capture Technology (PACT) facilities in Sheffield in the United Kingdom. TCM’s contribution to the ALIGN-CCUS project is aimed at bridging knowledge gaps as well as reducing HSE, technical and financial risks of technology upscaling.
In the ALIGN-CCUS 2019-2020 campaign at TCM, flue gas from Equinor’s combined cycle gas turbine (CCGT) plant in Mongstad was treated with the aqueous 2-amino-2-methyl-1-propanol (AMP) / piperazine (PZ) (CESAR 1) aqueous solvent. The CESAR 1 solvent was selected due to its lower energy consumption and higher stability as compared to monoethanolamine (MEA). Its promising characteristics have made CESAR 1 the new benchmark IEAGHG amine solvent. Emission control and monitoring as well as solvent consumption were among the study topics of the ALIGN-CCUS 2019-2020 campaign. The main goal of the campaign was to demonstrate that this advanced amine solvent can be used at a large scale and with a real flue gas. Results from the ALIGN-CCUS project are in the open domain, thus offering the opportunity to show, for the first time, qualitative and quantitative results on the measurement of AMP and PZ in the absorber effluent. Emission data will be presented in a companion paper.
In this work, we will present the chemical-analytical methods used for carrying out the emission measurements during the ALIGN-CCUS 2019-2020 campaign and present some of the results along with the lessons learned from this exercise.
2. Methods
2.1 Overview of the campaign and overall system description
The ALIGN-CCUS campaign at TCM was conducted from September 12th 2019 to January 10th 2020. As already stated above, flue gas from a CCGT plant was treated with the CESAR 1 solvent. Figure 1 is a sketch of the analytical set-up used for emission monitoring. Table 1 gives an overview of the analytical methods used for emission monitoring, their time of deployment, the measurement frequency and the compounds measured.

Table 1. Overview of the analytical methods used for emission monitoring, their time of deployment, the measurement frequency and the compounds measured.
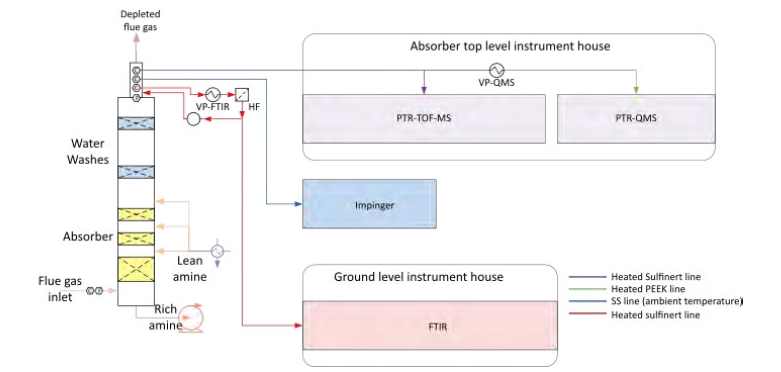
Fig. 1. Process flow diagram of emission sampling of absorber outlet. (VP: vaporizer).
2.2 Proton-Transfer-Reaction Mass Spectrometry (PTR-MS)
PTR-MS is a well-established technique for monitoring organic trace gases online (i.e., without sample pre- treatment), at a typical measurement frequency of 1 Hz and down to ppt levels [7]. In PTR-MS, the gas to be analyzed is introduced into a low-pressure reaction cell wherein organic molecules are softly ionized via gas-phase proton transfer reactions with H3O+ ions [8]. The protonated analyte molecules are detected in a quadrupole mass spectrometer (QMS) or a time-of-flight mass spectrometer (TOF-MS). [5] have described the use of PTR-MS for monitoring amines in the treated flue gas emanating from an amine-based PCCC plant. For the work presented herein, we used two different PTR-MS instruments, a PTR-TOF-MS analyzer (model PTR-TOF 8000; Ionicon Analytik, Innsbruck, Austria) and a PTR-QMS instrument (model QMS 300; Ionicon Analytik, Innsbruck, Austria). The latter is a cheaper and easier to use instrument version, which was conceived for industrial monitoring purposes. Both instruments were placed in an analyzer house on top of the absorption tower, and connected to the stack via a ~10 m long heated (120 °C) sampling line made of SilcoNert2000®/Sulfinert. The subsampling line of the PTR-QMS instrument included a vaporizer (VP-QMS) for evaporating potential mist particles. The operating parameters of both PTR-MS instruments were as follow: drift tube pressure 2 mbar, drift tube temperature 120 °C, drift tube voltage 500 V. The resulting reduced electric field strength (E/N) was ~150 Td (1 Td = 10-17 V.cm2).
Both PTR-MS instruments were calibrated for AMP and PZ using a HovaCAL® calibration gas generator (model
312-MF; IAS GmbH, Oberursel, Germany). A quantitative aqueous amine solution (AMP, PZ) was prepared by TCM’s analytical laboratory. This solution was evaporated at 180 °C in the HovaPOR evaporator (IAS GmbH, Oberursel, Germany) under a pure nitrogen atmosphere. By increasing the calibration solution flow into the evaporator, the humidity increases in the resulting calibration gas. This allowed us to study the response of the two PTR-MS instruments at different humidity levels.
2.3 Fourier Transform Infrared (FTIR) Spectroscopy
The FTIR instrument (Analect 5000; Schneider Electric) was located in the analyzer house at the bottom of the absorption tower. It was connected to the stack via a 100 m long sampling line made of SilcoNert2000®/Sulfinert. The flue gas was extracted from the stack at a fixed flow rate using a fast flow loop system mimicking isokinetic conditions [9]. The fast flow loop system included a vaporizer (VP-FTIR) for evaporating potential mist particles and a heated filter (HF). The temperatures of these units were adjusted to minimize the thermal degradation of analytes. The FTIR instrument was calibrated using the same HovaCAL® calibration gas generator as described above. Principal component regression (PCR) models were used for extracting CO2, H2O, NO, NO2, SO2, NH3, acetaldehyde, formaldehyde, AMP and PZ from the FTIR spectra.
2.4 Impinger sampling followed by Ion Chromatography Mass Spectrometry (IC-MS) analysis
Samples were extracted isokinetically and collected in a standard impinger sampling train including a condensation flask, a filter flask and three absorption flasks. Typical sampling times were 1-2 hours. The samples were analyzed in TCM’s laboratory using a Dionex Integrion HPIC System (model ICS-5000, Thermo Fisher Scientific) which included an IonPac CS19 column and an IonPac CG19 guard column.
3. Results and Discussion
3.1 Measurement of AMP emissions and encountered difficulties
The only compound detected by all four measurement techniques was AMP. The results obtained with the four methods were in good agreement. As shown in Figure 2, the distribution of the measured AMP volume mixing ratios was very similar, with the PTR-TOF-MS, the PTR-QMS and the FTIR instrument measuring median AMP levels of 433, 400 and 388 ppb, respectively. Mean measured AMP levels were 592, 549 and 503 ppb, respectively. Figure 3 shows an exemplary time series of AMP as recorded over a period of ten days. Two impinger samples were collected during this period. The measurements are again in good agreement, the only major discrepancy being the systematically lower levels measured by the FTIR instrument during periods with enhanced emissions. Table 2 shows a systematic comparison for all data collected during twelve impinger sampling periods, with sample collection times ranging from 42 to 136 minutes. The overall agreement was good, even though in some case large discrepancies were observed. It is not clear why the level of agreement varied between the samples. Future work is needed for addressing this issue.
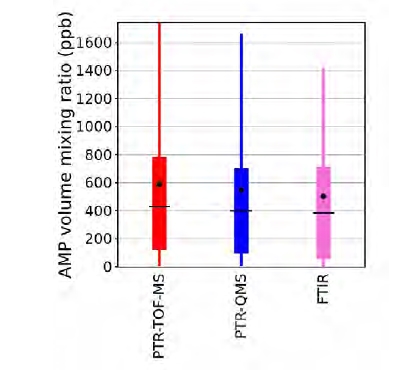
Fig. 2. Distribution of AMP volume mixing ratios as measured in the emitted flue gas by the PTR-TOF-MS, PTR-QMS and FTIR instruments, respectively, over the duration of the whole 2019-2020 ALIGN-CCUS campaign. Boxes represent 25th and 75th percentiles, the black line is the median, and the black dot is the mean. Whiskers represent 5th and 95th percentiles.
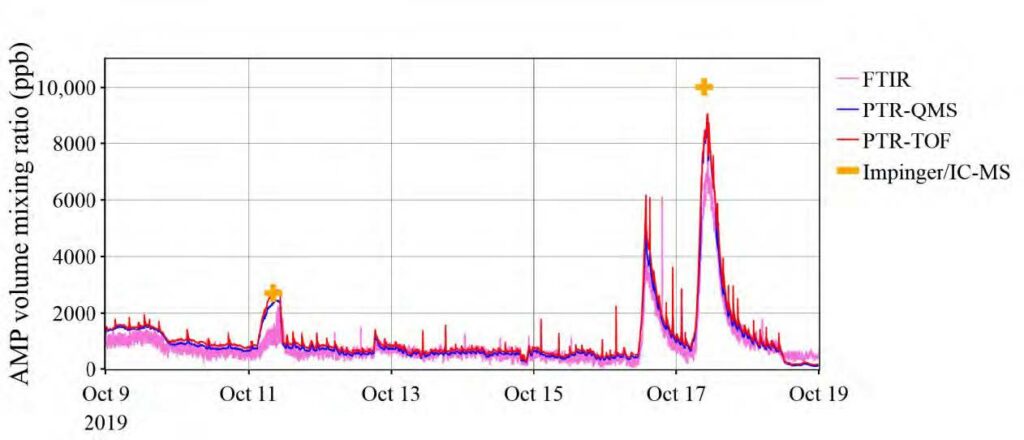
Fig. 3. Exemplary time series of AMP volume mixing ratios as measured in the emitted flue gas by the PTR-TOF-MS, PTR-QMS and FTIR instruments, respectively, in the period from October 9 to October 19, 2019. Also included are the two discrete measurement points obtained via impinger sampling followed by IC-MS analysis.
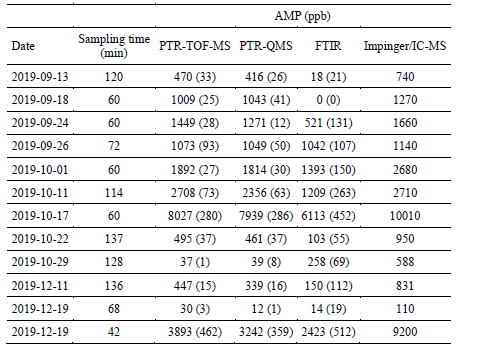
Table 2. AMP mixing ratios as measured by all four measurement techniques during the impinger sampling periods. Standard deviations are given in brackets.
A number of problems and difficulties were observed for all of the measurement methods used during the ALIGN- CCUS campaign. A forthcoming paper will address these issues in more detail and only a brief outline is given here.
The FTIR analyzer was sampling through a 100 m long sampling line. Long sampling lines are known to require long conditioning times for amines, meaning that peak levels were dampened and thus underestimated. In addition, we found that a stainless steel (SS) line originally connected to the FTIR instrument was corroded. The SS line was replaced with a sampling line made of polytetrafluoroethylene (PTFE) during the campaign. AMP degrades when in contact with a hot SS surface. It was thus important to reduce the temperature of the vaporizer (see Figure 1) to a level at which no AMP decomposition is observed. Finally, it should be noted that the AMP concentrations in the treated flue gas were close to the detection limit of the FTIR instrument, which made the measurements with this instrument less accurate.
The response of PTR-MS instrument to amines usually does not depend on the humidity of the sample matrix. This was not the case for AMP. The response of the PTR-QMS and the PTR-TOF-MS instruments varied by a factor of 5 between dry and humid conditions. For obtaining accurate results, it was essential to calibrate both instruments over the full range of humidity levels observed in the flue gas stack. Also, the reduction, processing and analysis of the PTR-TOF-MS data was highly complex and time-consuming and required a full time engagement of an expert scientist.
Impinger sampling followed by ICMS analysis is time consuming and laborious. Only 12 samples were thus collected and analysed over a period of the whole campaign. In addition, the measurement uncertainty was high at low ppb levels. Only AMP (typically >100 ppb) was thus quantitatively reported from the IC-MS analysis.
Finally, it should be noted, that during the ALIGN-CCUS campaign no mist was observed in the treated flue gas. Measurement problems that occurred in the presence of mist will be presented in a separate study.
3.2 Measurement of PZ
PZ was only present at low ppb levels in the treated flue gas, meaning that only the PTR-TOF-MS instrument was able to quantitatively detect it. PZ also exhibited a humidity-dependent response. It was thus necessary to carry out a humidity-dependent calibration of the PTR-TOF-MS instrument. The PTR-QMS instrument also detected an ion signal at m/z 87, which corresponds to protonated PZ. An intercomparison with the PTR-TOF-MS data revealed that a second peak at m/z 87 (which can only be resolved by the PTR-TOF-MS and not by the PTR-QMS) becomes quantitatively relevant at low ppb levels. Since these were typical emission levels during the ALIGN-CCUS 2019- 2020 campaign at TCM, it was not possible to quantitatively measure PZ with the PTR-QMS instrument. Impinger sampling followed by offline Ion Chromatography Mass Spectrometry (IC-MS) analysis suffered from a high measurement uncertainty at low ppb levels, meaning that not quantitative PZ data were reported during the ALIGN- CCUS 2019-2020 campaign.
3.3 Measurement of solvent degradation products
The PTR-TOF-MS analyzer also detected a series of solvent degradation products. The high mass accuracy (typically <10 ppm) of the measurement combined with an isotopic pattern analysis allowed us to identify the elemental composition (i.e., the molecular sum formula) of the decomposition products. Table 3 lists the m/z of the main signals detected in the flue gas, the assigned molecular sum formula and the name of the compound that we assigned (or tentatively assigned) to this signal based on previous work specified in the fourth column. An identification of compounds with a higher degree of confidence warrants complementary analyses by GC-MS or LC- MS.
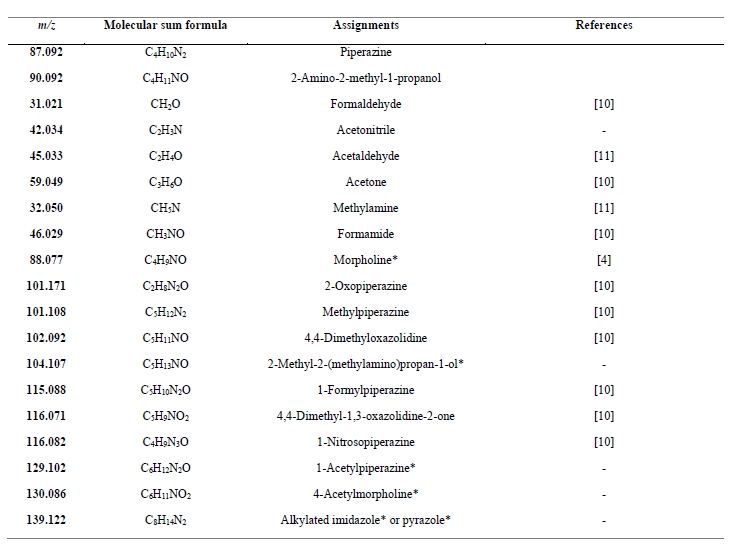
Table 3. Main m/z signals detected by the PTR-TOF-MS instrument in the flue gas that was emitted to the atmosphere when the amine plant was operated with the CESAR 1 solvent. The molecular sum formula was assigned unambiguously; the assignment to a specific chemical substance was based on chemical plausibility and literature data specified in the “References” column. Assignments marked with an asterisk are tentative.
Conclusions
Amine-based PCCC plants emit a variety of organic chemicals into the atmosphere. Treatment of CCGT flue gas with the CESAR 1 solvent generates ppb-to-ppm levels of AMP in the emission stream. Our study has shown that such emissions can be measured with sophisticated chemical-analytical techniques (PTR-TOF-MS; impinger sampling/IC-MS) but also with less demanding methods that are suitable for routine industrial monitoring purposes (PTR-QMS, FT-IR). Special care must be taken to avoid losses (due to adsorption and/or thermal decomposition of AMP) in the inlet system and to calibrate the online analyzers. Only the PTR-ToF-MS instrument was capable of detecting PZ at low ppb levels, which were typical emission levels during the ALIGN-CCUS campaign. The PTR- TOF-MS analyser is also capable of detecting amine degradation products, 17 of which were observed at significant levels in the flue gas after treatment with the CESAR 1 solvent. The unambiguous identification of these degradation products would, however, require complementary analyses using highly specific offline GC/LC-MSn methods.
While it seems unfeasible to make similar chemical-analytical efforts for emission characterization at each amine- based PCCC plant, TCM and its partners provide the know-how and infrastructure to characterize the emission profile of new solvents.
Acknowledgements
ACT ALIGN-CCUS Project No 271501. This project has received funding from RVO (NL), FZJ/PtJ (DE), Gassnova (NO), UEFISCDI (RO), BEIS (UK) and is co-funded by the European Commission under the Horizon 2020 programme ACT, Grant Agreement No 691712 (www.alignccus.eu).
The authors gratefully acknowledge the staff of TCM DA, Gassnova, Equinor, Shell and TotalEnergies for their contribution and work at the TCM DA facility. The authors also gratefully acknowledge Gassnova, Equinor, Shell, and Total as the owners of TCM DA for their financial support and contributions.
References
- SEPA Scottish Environment Protection Agency. Review of amine emissions from carbon capture systems. Stirling, UK, pages 1–86, 2015.
- Nielsen CJ, Herrmann H, and Weller C. Atmospheric chemistry and environmental impact of the use of amines in carbon capture and storage (ccs). Chem. Soc. Rev., 41 :6684–6704, 2012.
- Låg M, Lindeman B, Instanes C, Brunborg G, and Schwarze P. Health effects of amines and derivatives associated with CO2 capture. 05 2011.
- Morken A, Pedersen S, Kleppe ER, Wisthaler A, Vernstad K, Ullestad Ø, Flø NE, Faramarzi L, and Hamborg ES. Degradation and emission results of amine plant operations from MEA testing at the CO2 technology centre mongstad. Energy Procedia, 114 :1245–1262, 07 2017.
- Zhu L, Schade G, and Nielsen CJ. Real-time monitoring of emissions from monoethanolamine-based industrial scale carbon capture facilities. Environmental science & technology, 47, 11 2013.
- Zhu L, Mikoviny T, Morken AK, Tan W, and Wisthaler A. A compact and easy-to-use mass spectrometer for online monitoring of amines in the flue gas of a post-combustion carbon capture plant. International Journal of Greenhouse Gas Control, 78 :349 – 353, 2018.
- Lindinger W, Hansel A, and Jordan A. On-line monitoring of volatile organic compounds at pptv levels by means of proton-transfer-reaction mass spectrometry (PTR-MS) medical applications, food control and environmental research. International Journal of Mass Spectrometry and Ion Processes, 173(3) :191 – 241, 1998.
- Hansel A, Jordan A, Holzinger R, Prazeller P, Vogel W, and Lindinger W. Proton transfer reaction mass spectrometry: on-line trace gas analysis at the ppb level. International Journal of Mass Spectrometry and Ion Processes, 149-150 :609 – 619, 1995. Honour Biography David Smith.
- Shah MI, Lombardo G, Fostås B, Benquet C, Morken AK, and de Cazenove T. CO2 capture from RFCC flue gas with 30w% MEA at Technology Centre Mongstad, process optimization and performance comparison. In 14th Greenhouse Gas Control Technologies Conference Melbourne, pages 21–26, 2018.
- Wang T. Degradation of Aqueous 2-Amino-2-methyl-1-propanol for Carbon Dioxide Capture. PhD thesis, 2013.
- Cuccia L, Bekhti N, Dugay J, Bontemps D, Louis-Louisy M, Morand T, Bellosta V, and Vial J. Monitoring of the blend 1- methylpiperazine/piperazine/water for post-combustion CO2 capture. Part 1: Identification and quantification of degradation products. International Journal of Greenhouse Gas Control, 76:215 – 224, 2018.
A compact and easy-to-use mass spectrometer for online monitoring of amines in the flue gas of a post-combustion carbon capture plant (2018)
Liang Zhua, Tomáš Mikovinya, Anne Kolstad Morkenb, Wen Tana, Armin Wisthalera,⁎
aDepartment of Chemistry, University of Oslo, P.O. Box 1033, 0315, Oslo, Norway bTechnology Centre Mongstad (TCM), 5954, Mongstad, Norway ⁎ Corresponding author
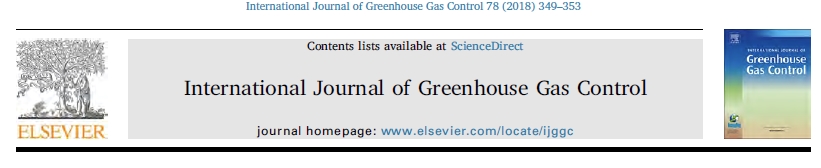
Abstract
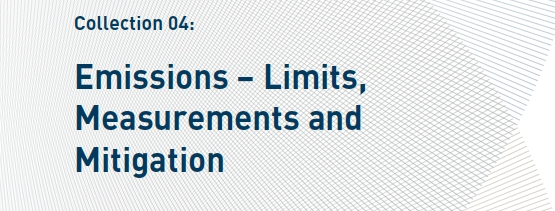
We herein report on the adaptation and deployment of a compact and easy-to-use mass spectrometer for online monitoring of amines in industrial flue gas at ppb to ppm levels. The use of ammonia as a source gas in proton- transfer-reaction mass spectrometry (PTR-MS) greatly simplifies the detection of amines, making it possible to use a low-end commercial instrument version (PTR-QMS 300) for the measurements. We characterized the analytical performance of the instrument (sensitivity, limit of detection, precision, matrix effects) for nine sol- vent amines (monoethanolamine, dimethylaminoethanol, aminomethylpropanol, methyldiethanolamine, di- glycolamine, piperazine, aminoethylpiperazine, methylpiperazine, N-(2-hydroxyethyl)piperazine) and three degradation amines (methylamine, dimethylamine, trimethylamine). The new analyzer was tested and validated in side-by-side measurements with established emission monitoring techniques at the Technology Centre Mongstad (TCM) in Norway. After validation, the instrument was permanently installed on top of the absorber tower to deliver real-time amine emission data to the plant information management system.
This article is behind a paywall. Futher information: https://www.sciencedirect.com/science/article/abs/pii/S1750583618304122?via%3Dihub
Ambient Measurements of Amines by PTR-QiTOF: Instrument Performance Assessment and Results from Field Measurements in the Vicinity of TCM, Mongstad (2016)
Tomáš Mikovinya, Claus J. Nielsena, Wen Tana, Armin Wisthalera*, Liang Zhua, Anne Kolstad Morkenb, Terje Niøten Nilsenb
aDepartment of Chemistry, University of Oslo, P.O. Box 1033 Blindern, 0315 Oslo, Norway bCO2 Technology Centre Mongstad (TCM DA), 5954 Mongstad, Norway *Corresponding author
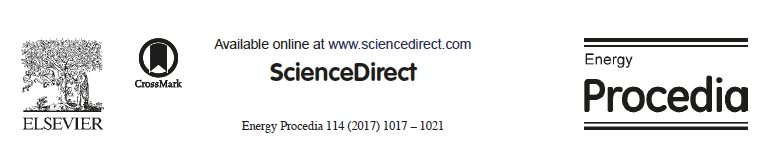
© 2017 The Authors. Published by Elsevier Ltd. This is an open access article under the CC BY-NC-ND license. Peer-review under responsibility of the organizing committee of GHGT.
Abstract
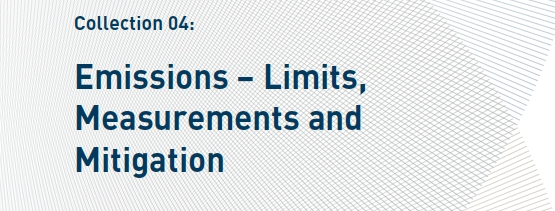
A PTR-QiTOF instrument was deployed in the field in the vicinity of the CO2 Technology Center Mongstad (TCM) for measuring amines in ambient air. The observed single-to-double-digit pptv levels of dimethylamine (DMA) and trimethylamine (TMA) are comparable to natural background values reported in the literature for these species. No indication was found that these small alkylamines stem from industrial activities at Mongstad. Monoethanolamine (MEA) was observed at single-digit pptv levels, but further analytical work is needed to validate measurements at such low concentrations. No indication was found that small enhancements in ambient MEA levels were caused by emissions from TCM. No other industrial amines (2-amino-2- methylpropanol, diethylamine and piperazine) were detected in ambient air. According to the current state of knowledge, the observed levels of amines pose no harm to human health or the environment.
1. Introduction
Amine-based capture of carbon dioxide (CO2) results in the release of trace amounts of amines to the atmosphere. While amines are nowadays routinely monitored in stack gas, the detection of amines under highly diluted atmospheric conditions remains an analytical challenge [1]. Proton-Transfer-Reaction Mass Spectrometry (PTR-MS) has been successfully used for on-line monitoring of amines in stack gas [2,3] and in atmosphere simulation chambers [4,5,6]. Recently, the ultra-sensitive proton-transfer-reaction quadrupole ion guide time-of-flight mass spectrometer (PTR-QiToF-MS) has become available [7], which is capable of detecting trace gases at single-digit pptv levels (1 pptv = 1 pmol mol-1 = 10-12 v/v). In the present study, we have used this novel analytical tool for carrying out exploratory measurements of amines in ambient air in the vicinity of the CO2 Technology Centre Mongstad (TCM).
2. Methods
Ambient air measurements were carried out at Sunsbø (60°46’10.1″N, 5°09’08.6″E), Sande (60°50’56.6″N, 5°00’21.0″E) and Mongstad West (60°48’45.7″N, 5°00’43.4″E) from Aug 6 – 28, Aug 28 – Sept 10 and Sept 10 – 25, 2015, respectively. The geographic location of the three measurement sites and of TCM is shown in Figure 1.
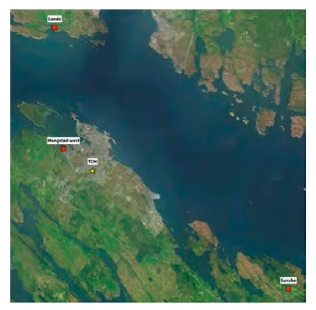
Fig. 1. Geographical map showing the locations of the measurement sites at Sunsbø, Sande and Mongstad West and of the Technology Center Mongstad (TCM).
PTR-MS is an on-line chemical ionization (CI) technique for atmospheric trace gases that has been routinely deployed in atmospheric chemistry field studies over the past decade [8]. Only recently, the ultra-sensitive PTR- QiTOF-MS instrument version has become available which detects gaseous analytes at single-digit pptv-levels [7].
We operated the PTR-QiTOF instrument in the routine mode of operation recommended by the manufacturer (H3O+ CI; drift tube pressure 3.8 mbar, drift tube temperature 60 °C, reduced electric field strength 120 Td with 1 Td = 10-17 V cm2). Mass spectra were recorded in the m/z 15 to m/z 510 range, but only selected signals corresponding to protonated methylamine, dimethylamine (DMA) and ethylamine, trimethylamine (TMA), monoethanolamine (MEA), 2-amino-2-methylpropanol (AMP), diethylamine and piperazine, respectively, were analyzed in detail. The list of target amines was taken from a previous study [1]. The PTR-TOF Data Analyzer v4.44 was used for data analysis [9]. 2-minute and 1-hour averages were generated from the data output.
An optimized inlet system was used for minimizing inlet losses of amines. Ambient air was sampled at a flow rate of 12 liters per minute trough a passivated stainless steel tube (material: SilcoNert® 2000, outer diameter: 6.35 mm, length: 115 cm, temperature: 60 °C). The PTR-QiTOF instrument sub-sampled a flow of 0.5 liters per minute through a heated capillary (material: PEEK, outer diameter: 1.59 mm, temperature: 60 °C). No evidence for inlet losses was found when ambient air was spiked with known amounts of DMA and TMA at pptv levels.
Amine-free air generated from compressed and catalytically cleaned (Pt/Pd at 325 °C) ambient air was periodically (every 12 hours for 30 minutes) fed to the inlet system for instrumental background determination.
Instrumental response factors for DMA, TMA and MEA were calculated from ion-molecule reaction kinetics using the molecular properties reported previously [4,5,6]. The estimated accuracy of the reported volume mixing ratios is ±20% for volume mixing ratios above 10 pptv.
TCM is part of a large industrial complex (refinery, power plant, oil terminal) at Mongstad which is located in a pristine coastal environment. Oil and gas tracers were used for distinguishing between periods when the outflow from the Mongstad complex was advected to the sampling sites and periods when natural background air was sampled. TCM was in operation during the ambient air measurement campaign, with MEA being used for CO2 capture.
3. Results
AMP, diethylamine and piperazine were not detected at levels above 10 pptv in the recorded mass spectra. It was not possible to measure these species at single-digit pptv levels due to mass spectral interferences. The PTR-QiTOF instrument, in its routine mode of operation, was not capable of detecting methylamine. The abundant O + signals distorted the mass spectrum in the region where methylamine is detected.
The hourly average time series of DMA, TMA and MEA are shown in Figures 2a, 2b and 2c, respectively. The color-coded data points identify the time periods when the outflow from the Mongstad industrial complex was advected to the sampling site.
DMA was observed at single-digit pptv levels, which is at or close to the instrumental detection limit. The detection of amines at such low concentrations is an analytical challenge and all single-digit pptv data reported herein should be taken with caution. More analytical validation work is needed in this concentration regime. The 2- minute average data show episodic short-term enhancements in the 10 to 22 pptv range at all three measurement sites. These enhancements were found both in natural background air and in the outflow from the Mongstad complex.
TMA levels were typically close to zero, with the exception of an episode lasting from Sept 1 to 12 when hourly average volume mixing ratios up to 49 pptv were recorded. Enhanced levels of TMA were observed at the Sande and the Mongstad West site, both in natural background air and in the outflow from the industrial site.
MEA levels were also in the single-digit pptv range. Yet again, these concentrations should be interpreted with caution. A 1-2 pptv enhancement was observed in the period from Aug 31 to Sept 10, both in natural background air and in the Mongstad outflow. This comes as a surprise as MEA is not believed to have natural sources. Further research is warranted to confirm these findings and exclude potential signal interferences for MEA.
The time series data indicate that amine levels were not enhanced in the Mongstad outflow as compared to natural background conditions. This is also reflected in the overall statistical analysis summarized in Table 1.
4. Discussion and Conclusions
A PTR-QiTOF instrument was successfully deployed in the field for the first time to measure amines in ambient air. The observed single-to-double-digit pptv levels of DMA and TMA are comparable to coastal background values reported in the literature for these species [10]. No indication was found that these small alkylamines stem from industrial activities at Mongstad. According to the current state of knowledge, the observed levels of alkylamines pose no direct or indirect harm to human health or the environment.
TCM was operating on MEA during the ambient measurements. Single-digit pptv levels of MEA were observed in ambient air, but no indications were found that these small enhancements were caused by emissions from TCM. Further analytical work is, however, needed to validate MEA measurements at such low concentrations. No other industrial amines (AMP, diethylamine and piperazine) were detected.
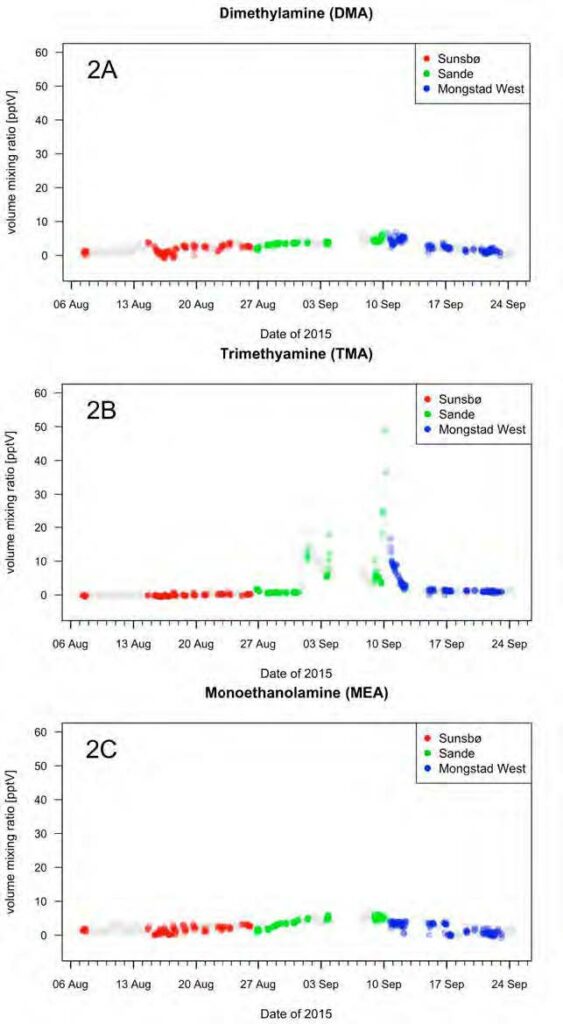
Fig. 2. Hourly average time series of DMA, TMA and MEA amine as measured at Sunsbø, Sande and Mongstad West in August and September of 2015. The color-coded data points identify the time periods when the Mongstad outflow was advected to the sampling sites.
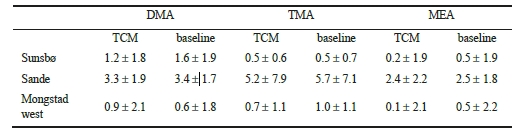
Table 1. Average mixing ratios (in pptv) of DMA, TMA and MEA as observed at the Sunsbø, Sande and Mongstad West sites, respectively, when the Mongstad plume was advected and under natural background conditions. No statistically significant enhancement of amines was found in the outflow from the Mongstad industrial complex as compared to natural background conditions.
References
- Vik AF, Rostkowski P. Amines in ambient air at TCM – Monitoring program May 2012 – April 2013, NILU Report OR 31/2015 (2015)
- Zhu L, Schade GW, Nielsen CJ. Real time monitoring of emissions from monoethanolamine-based industrial scale carbon capture facilities. Environ Sci Technol. 2013; 47, 14306-14314
- Morken AK, Nenseter B, Pedersen S, Chhaganlal M, Feste JK, Tyborgnes RB, Ullestad Ø, Ulvatn H, Zhu L, Mikoviny T, Wisthaler A, Cents T, Bade OM,GKnudsen J, de Koeijer G, Falk-Pedersen O, Hamborg ES. Emission results of amine plant operations from MEA testing at the CO2 Technology Centre Mongstad. Energy Procedia 2014; 63, 6023–6038
- Nielsen CJ, D´Anna B, Bossi R, Bunkan AJC, Dithmer L, Glasius M, Hallquist M, Hansen AMK, Lutz A, Salo K, Maguta MM, Nguyen Q, Mikoviny T, Müller M, Skov H, Sarrasin E, Stenstrøm Y, Tang Y, Westerlund J, Wisthaler A. Atmospheric Degradation of Amines (ADA). Summary report from atmospheric chemistry studies of amines, nitrosamines, nitramines and amides, University of Oslo, Oslo, Norway 2012; ISBN 978-82-992954-7-5, http://urn.nb.no/URN:NBN:no-30510
- Nielsen CJ, D’Anna B, Karl M, Aursnes M, Boreave A, Bossi R, Bunkan AJC, Glasius M, Hansen AMK, Hallquist M, Kristensen K, Mikoviny T, Maguta MM, Müller M, Nguyen Q, Westerlund J, Salo K, Skov H, Stenstrøm Y, Wisthaler A. Atmospheric Degradation of Amines (ADA). Summary report: Photo-oxidation of methylamine, dimethylamine and trimethylamine, NILU Report OR 2/2011
- Nielsen CJ, D’Anna B, Dye C, George C, Graus M, Hansel A, Karl M, King S, Musabila M, Müller M, Schmiedbauer N, Stenstrøm Y, Wisthaler A. Atmospheric Degradation of Amines (ADA). Summary report: Gas phase photo-oxidation of 2-aminoethanol (MEA), NILU Report OR 8/2010
- Sulzer P, Hartungen E, Hanel G, Feil S, Winkler K, Mutschlechner P, Haidacher S, Schottkowsky R, Gunsch D, Seehauser H, Striednig M, Jürschik S, Breiev K, Lanza M, Herbig J, Märk JL, Märk TD, Jordan A. A Proton Transfer Reaction-Quadrupole interface Time-Of-Flight Mass Spectrometer (PTR-QiTOF): High speed due to extreme sensitivity. Int J Mass Spectrom 2014; 368:1–5
- De Gouw J, Warneke C. Measurements of volatile organic compounds in the earth’s atmosphere using proton-transfer-reaction mass spectrometry, Mass Spectrom Rev 2007; 26, 2:223–257
- Müller M, Mikoviny T, Jud W, D’Anna B, Wisthaler A. A new software tool for the analysis of high resolution PTR-TOF mass spectra, Chemom Intell Lab Syst 2013; 127:158–165
- Ge X, Wexler AS, Clegg SL. Atmospheric amines – Part I. A review, Atmos Environ 2011; 45:524-546
Emission results of amine plant operations from MEA testing at the CO2 Technology Centre Mongstad (2014)
Anne K. Morkena,b, Bjarne Nenseterb, Steinar Pedersenb, Milan Chhaganlala,b, Jane K. Festea,b, Rita Bøe Tyborgnesa,b, Øyvind Ullestada,b, Helge Ulvatna,b, Liang Zhuc, Tomas Mikovinyc, Armin Wisthalerc, Toine Centsa,d, Otto M. Badee, Jacob Knudsene, Gelein de Koeijerb, Olav Falk-Pedersena,f, Espen S. Hamborga,b,*
aCO2 Technology Centre Mongstad (TCM DA), 5954 Mongstad, Norway bStatoil ASA, P.O. Box 8500, 4035 Stavanger, Norway cUniversity of Oslo, Department of Chemistry, P.O. Box 1033 Blindern, 0315 Oslo, Norway dSasol Technology, PO Box 5486, Johannesburg 2000, South Africa eAker Solutions, P. O. Box 222, 1326 Lysaker, Norway fGassnova SF, Dokkvegen 10, 3920 Porsgrunn, Norway *Corresponding author
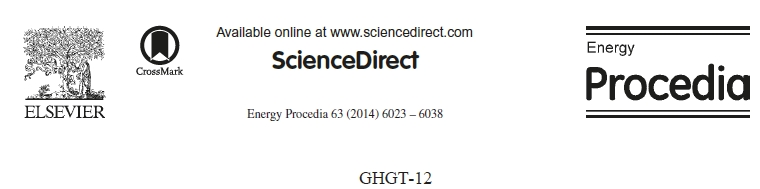
doi: 10.1016/j.egypro.2014.11.636
Abstract
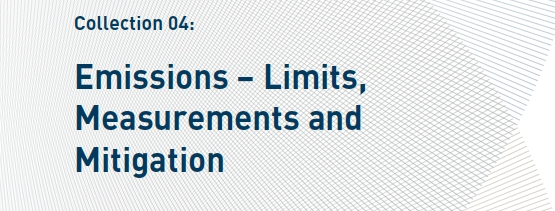
Extensive atmospheric emission monitoring has been conducted at the CO2 Technology Centre Mongstad (TCM DA) during amine based post-combustion CO2 capture. The TCM DA amine plant was operated with an aqueous monoethanolamine (MEA) solvent system, treating flue gas from a combined heat and power (CHP) plant. Emission monitoring was conducted by a Fourier Transform Infrared (FTIR) Spectroscopy analyzer, a Proton Transfer Reaction Time-of-Flight Mass Spectrometry (PTR-TOF- MS) analyzer, and manual isokinetic sampling followed by off-line analysis in the laboratory.
Atmospheric emissions of MEA were very low throughout the entire campaign, ranging from a few to a few hundred parts per billion (ppb, 1 ppb = 10-9 v/v). Atmospheric emissions of MEA amine based degradation products such as nitrosamines and nitramines were below detectable levels. Atmospheric emissions of ammonia (NH3) were in the low ppm range. Methylamine was emitted at low ppb range.
Absorber wash water sections were found to effectively reduce atmospheric emissions from amine based solvent system.
1. Introduction
The CO2 Technology Centre Mongstad (TCM DA) has in collaboration with partners undertaken several months test using the non-proprietary aqueous monoethanolamine (2-aminoethanol, MEA) solvent system at 30 wt% and 40 wt% in an attempt to characterize the performance and atmospheric emissions from such operations [1,2,3]. The operations were carried out at a considerably large scale of about 50.000 Sm3/h of flue gas supply flow rates from a combined heat and power (CHP) plant, as described elsewhere [1,2,3]. In the CHP plant, the natural gas is combusted in a gas turbine and the flue gas content and characteristics are similar to those of a combined cycle gas turbine (CCGT) power plant. TCM DA has made significant investment in equipment and instrumentation for monitoring of stack emissions. Continuous efforts are being done to improve sampling methods, sampling lines and the instrumental analysis.
Quantitative emission data from a representative CO2 capture plant is one remaining knowledge gap in the assessment of health and environmental risks posed by the amine-based post combustion capture (PCC) technology [4]. A health risk analysis for the emissions to air from the amine plant TCM DA was recently published [5]. The emission permit granted to TCM DA by the Norwegian Environmental Agency (Miljødirektoratet) in November 2011 regulates the emission levels for solvent amines, alkylamines, aldehydes and ammonia [6]. It also sets requirements for online monitoring and how to calculate the nitrosamine and nitramine environmental concentrations by a dispersion calculation method. The air and drinking water concentrations of 0.3 ng/m3 and 4 ng/L respectively were associated with negligible excess risk level for cancer (10-6) after lifelong exposure to nitrosodimethylamine (NDMA). Since all amines that are emitted to air from the absorber stack may undergo photo-oxidation in the atmosphere and be converted to nitramines or nitrosamines they will contribute to the environmental concentrations as calculated by the dispersion simulation method. In the granted permit the total sum of nitrosamines and nitramines must be below the given limits. Therefore both amine emissions and direct emissions of nitrosamines and nitramines will contribute to the total environmental budget of the harmful compounds.
Although sampling and analysis of flue gases in general are well known, the wet flue gas containing solvent amine, amine degradation products and other trace components give many sampling and analytical challenges. Very limited standard methods are established for such a task. Several studies were undertaken by international experts for the, now terminated, Carbon Capture Plant Mongstad (CCM) project, and much of the work is available for the public [7]. The CCM project developed a toolbox for qualifying amine based solvent technologies, consisting of the steps liquid sampling, isokinetic gas sampling, sample preservation and sample logistics, sample work-up and analytical procedures, atmospheric chemistry including dry and wet deposition, dispersion modelling including local Mongstad weather conditions, toxicology assessment of major degradation products as nitramines and nitrosamines, solvent degradation rig and test protocol for solvent stress testing as well as process emission reducing technologies. The analytical measurement chain was essential in the toolbox and it is also the basis for the current work.
This work is part of a continuous effort of gaining better understanding of the performance potential of the non- proprietary aqueous MEA solvent system, conducted by TCM DA and its affiliates and owners, in order to test, verify, and demonstrate CO2 capture technologies [1, 2, 3]. The purpose of the current work is to provide results which quantify the amounts and the compositions of atmospheric emissions sampled and analyzed during amine plant operations treating CHP flue gases. A thorough overview and discussion of available equipment and instrumentation for monitoring of stack emissions will be given. The results are believed to provide realistic emission figures for emission monitoring and control for any future large scale carbon capture and storage (CCS) project due to the considerable size of the TCM DA amine plant.
2. Instrument and analysis
A description of the TCM DA amine plant is given elsewhere [1,2,3].
2.1 Overall system description and instrument position
Removing CO2 from flue gas by using post-combustion amine based CO2 capture reduces the emission of greenhouse gases to the atmosphere, but inevitably causes some emissions of amines and amine related degradation products to the atmosphere. Thus, qualitative and quantitative analysis of the emitted components is very important, but this task is far from trivial. For practical purposes, analytical instruments are preferably placed at ground level, but in that case a long sampling line (often >50 m) is required to direct the treated flue gas from the top of the absorber into the apparatus at ground level. This sample line has to be heated to well above the dew point of the gas to avoid condensation and possibly unwanted adsorption and/or reaction of emitted components.
TCM DA applies different measurement techniques to monitor and quantify the amounts and concentrations of emitted compounds. Some of the analyzer techniques currently applied on a permanent basis are;
- Fourier Transform Infrared (FTIR) Spectroscopy
- Proton Transfer Reaction Time of Flight Mass Spectrometry (PTR-TOF-MS)
- Manual isokinetic sampling technique with impingers and subsequent off-line laboratory analysis (carried out by TCM DA, Statoil CP Laboratory, SINTEF and Ramboll)
Online gas phase concentration measurements are also performed at ground level (via a sample line) using a Fourier Transform Infra-Red (FTIR) Spectroscopy instrument and a Proton Transfer Reaction – Time of Flight – Mass Spectrometry (PTR-TOF-MS) device. This online equipment is placed in an analyzer house at ground level. At the absorber top, isokinetic sampling is performed on a regular basis. There is an analyzer house and a shelter on the top of the amine absorber where all the equipment is located, as seen in Figure 1.
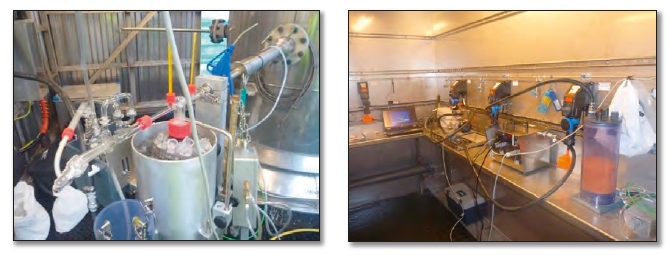
Figure 1: Emission sampling set-up on the top of the amine absorber. Stack configuration (left) and sampling control from analyzer house (right).
Extracted gas is sampled from the stack through an impinger train containing absorption liquids. By onsite measurement of the gas flow and laboratory analysis of the impinger liquids, the gas phase concentration of different components can be determined. The measurement system is shown schematically in Figure 2 and the techniques are further explained in the sections below.
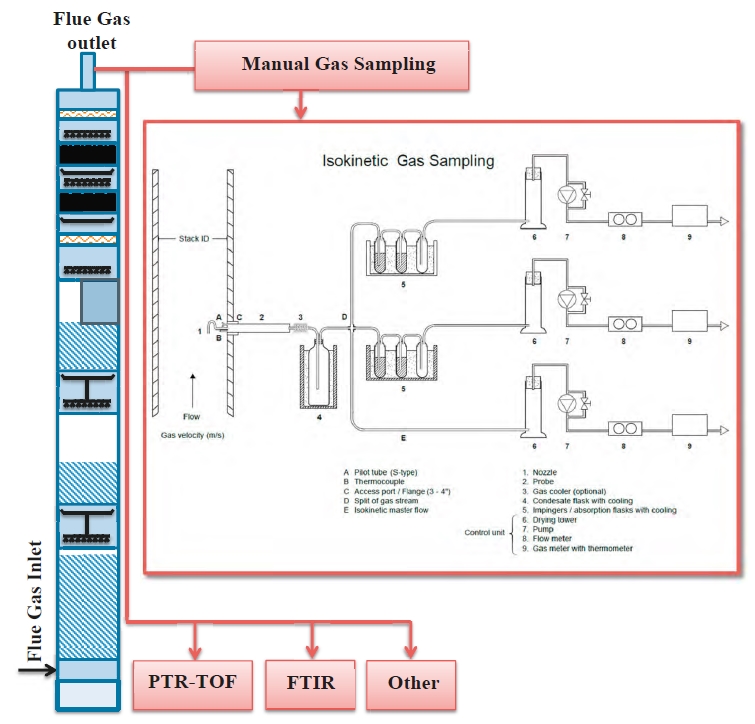
Figure 2: Schematic drawing of the emission monitoring set-up at the TCM DA amine plant.
2.2 Sampling lines
The sampling line bundle installed at TCM DA is 101 meters long. It consists of 3 separate lines made from the following materials, respectively:
- PFA Teflon®
- Electro-polished stainless steel
- Sulfinert®-treated passivated stainless steel
All lines can be heated to 140 °C. Sample transfer via a heated sampling line has several benefits over placing the equipment at the top of the absorber:
- Easy access to the analyzer for maintenance and calibration and to utilities such as power, gas supplies, etc.
- Increased physical space for the analyzer
- Safer operations
Some negative aspects are however:
- Delayed analyzer response
- Potential degradation reactions and adsorption effects in the sampling line
Potential sample line effects are rarely reported in open literature. It is generally accepted is that the sample path should be kept as short as possible, and that the line temperature should be well above the dew point. However, increasing the temperature too much may lead to unwanted decomposition, to potential formation of nitrosamines, and to other sampling artefacts. Switch between different sample lines should be avoided due to memory effects.
The effects of different sample lines were investigated by Cents et al [8].
- FTIR analyzer
The FTIR model Anafin 2000 is employed at TCM DA to measure standard gas phase components (CO2, water, NOx, SOx) as well as amines, aldehydes and ammonia. The analyzer operates at wave numbers between 500 and 7000 cm-1, with a resolution of 2 cm-1. The path length is 7 meters. The detection limit for amines, aldehydes and ammonia is on the order of 1 ppmv. According to the discharge permit from the Norwegian Environmental Agency (Miljødirektoratet), TCM DA is allowed to emit 6 ppmv of total amines as a daily average [6]. For this purpose, the detection limit of the FTIR instrument is satisfactory.
The FTIR is connected via heated sampling lines to sampling probes at the absorber inlet (downstream DCC), absorber outlet and desorber overhead condenser outlet. An automatic stream selector makes it possible to program the plant’s control system to alternate between the different measuring locations as desired. The FTIR is calibrated for a list of standard flue gas pollutants, including CO2, SO2, NH3, etc., as well as solvent amines and some volatile degradation products e.g. aldehydes. The instrument is not set up for measuring alkyl amines, nitrosamines and nitramines.
The FTIR technique has the advantage that the sample is measured without any preconditioning, hence reducing the risk for analytical artefacts. To avoid water condensation, the FTIR gas cell is heated to 85°C and the sampling lines are heated to 120°C. Target compounds contained in mist or droplets are likely to be evaporated at these temperatures. The FTIR monitor thus measures the total content of analytes in the flue gas. A draw-back of the FTIR technique is the interference from water vapor which results in a relatively high detection limit. The experience is that NH3 and amines can be detected down to 1 ppm levels. This is also in accordance with earlier measurements of gaseous emissions in post combustion carbon capture [9, 10].
2.3 PTR-TOF-MS analyzer
The PTR-TOF-MS (model PTR-TOF 8000) used is manufactured by Ionicon Analytik (Innsbruck, Austria). The PTR technique has been widely used for environmental volatile compound measurements for over a decade. Its measurement principle is based on soft ionization, via proton transfer, followed by high mass resolution mass spectrometric analysis. At TCM DA, the PTR-TOF-MS instrument subsamples from the main sample line through a heated (100-130°C) Siltek inlet line. The sample flow is diluted by a factor of 10 to 20 with bottled synthetic (zero) air, to avoid ion signal titration caused by high ammonia levels. The PTR-TOF-MS is able to measure amines, ammonia, aldehydes, ketones, carboxylic acids, nitramines and nitrosamines which are all important target compounds in amine based CO2 capture. The analytical setup at Mongstad is described in recent publications by Zhu et al. [11, 12].
2.4 Manual gas emission sampling
The analytical value chain applied for manual gas emission sampling and analysis is schematically shown in Figure 3. This value chain governs the measurement and is described in details below.
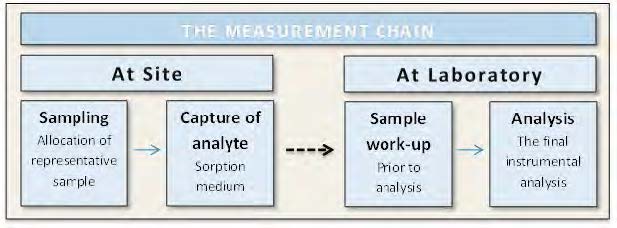
Figure 3: Schematic drawing of the emission measurement value chain.
Sampling. The TCM DA stack is designed to achieve flow conditions suitable for isokinetic flue gas sampling as specified in the standard EN 15259:2007. The stack is insulated to minimize condensation. Sampling nozzles are located at a level 2 meters below the stack exit. A sampling system from Paul Goethe GmbH in Germany is used for allocation of a gas emission sample. The equipment is operated from an associated control unit (iTES). The special sampling equipment configuration is assembled for amine emissions based on experiences from the CCM project [7]. Isokinetic gas sampling principles are used to secure representative sampling from a ducted gas stream where two-phase conditions (particles or droplets with diameter > 1 μm) are present or may occur. From an amine absorber the presence of droplets in the flue gas has to be considered, hence isokinetic gas sampling is an assurance for representative samples.
Capture of analyte. The double tube sampling probe was cooled with pressurized air in order to start condensation of the extracted gas sample stream. Typical amine emission analytes are captured by two principles, condensation and liquid absorption. It is experienced that the main sampling step is condensation. The condensate flask is kept cool in an ice bath and has a size and design to maximize the condensation capacity. In this way the gas is dried and further downstream split to subsequent impinger trains or solid adsorbents. It is further experienced that only for the most volatile components like NH3, small alkyl amines and aldehydes the second trap based on stepwise liquid absorption or solid phase adsorption is significant. In case of mist formation in the absorber, submicron aerosols will enter the sampling train. It is known that aerosols potentially can have limited retention through liquid sampling systems. In order to improve the capture of aerosols, a high capacity condensation step is followed by jet- impinger flasks to force agglomeration. However mist is normally not associated with the CHP flue gas.
The condensates were preserved with sulfamic acid at site directly after sampling to avoid potential nitrosation of secondary amines [7]. Ammonia and the different amines were absorbed in 0.05 M sulphuric acid, the aldehydes and ketones were adsorbed on DNPH cartridges (Sep-Pak DNPH-Silica Long Body Cartridges, Waters). For nitrosamines and nitramines 10 g/L sulfamic acid solutions were used as second sampling step.
Sample work-up. Samples were brought to TCM laboratory and immediately cooled or frozen for storage until analysis. As a principle the condensate sample was prepared for analysis first and subsequent absorbent solutions were prepared and analyzed secondly, with various experimental techniques (Table 1). This often includes extensive laboratory work.
Analysis. Expected degradation and emission products from a MEA based solvent system, were assessed from a recent study using the solvent degradation rig for stress testing of MEA [13]. The target analytes for the current work is given in Table 1. These compounds cover the requirements set forth by the Miljødirektoratet in the emission permit.
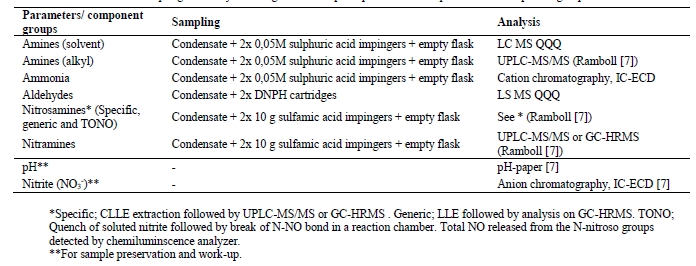
Table 1. TCM DA sampling and analysis configuration and principle for different parameters / component groups.
Amines, nitrosamines, and aldehydes were analyzed using an LC MS-MS QQQ (Agilent). The condensate from the first impinger was analyzed directly on the LC MS, the acidic impinger solutions were diluted before analysis.
Ammonia was analyzed on an ion chromatograph (IC).
2.6 Additional analyzer techniques: Voice200 and PTR-QMS
TCM DA also tested a Voice200 analyzer from SYFT Technologies and a PTR-QMS 300 analyzer from Ionicon.
These instruments operate on the same measurement principle as the PTR-TOF-MS but include cheaper and less specific quadrupole mass analyzers. Results from both analyzers compared well with the PTR-TOF-MS data. The results are not presented in this paper.
3. Results and Discussions
3.1 Analysis of Solvent samples
Solvent degradation processes were monitored during the course of the entire MEA campaign. The solvent amine, ammonia, and some degradation products were analyzed by TCM DA and Statoil CP laboratories. Alkyl amines, aldehydes, ketone, generic nitrosamines, solvent specific nitrosamines and nitramines were analyzed by Ramboll and SINTEF laboratories.
The concentration of the solvent amine was observed to remain stable over the extended period of the campaign
indicating reasonable degradation rates of the solvent amine. The main degradation products of MEA were found to be amides, amino acids and other amines. Heat stable salts were also measured through the entire campaign, anions (OA, GA, FA, NO3–) by IC and total heat stable salts (HSS) by ion exchange and titration. Figure 4 displays the evolution of various degradation products and heat stable salts in the solvent. The components and amounts found were expected from an aqueous based MEA solvent system [9].
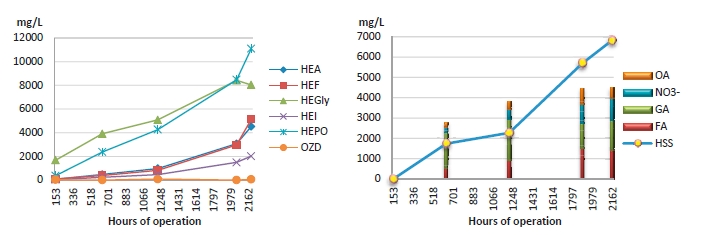
Figure 4: Results from some major degradation components (left figure) and heat stable salt formations (right figure) in the aqueous MEA solvent system during treatment of CHP flue gas.
Two solvent specific nitrosamines, N-nitrosodiethanolamine (NDELA) and N-nitroso-2-hydroxyethyl-glycine (Nitroso-HeGly), were detected in the solvent as the degradation process progressed (Figure 5). The total concentrations of nitrosamines (TONO) were measured to be 797 μmol/L.
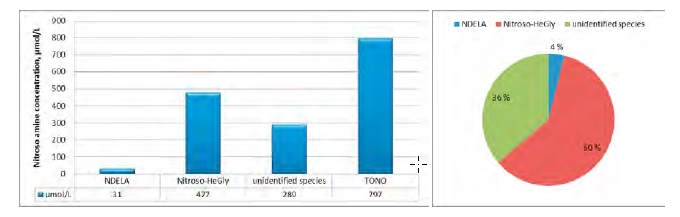
Figure 5: Results from degradation of solvent amine MEA (04.02.2014) [13].
Since MEA is a primary amine it is not expected to form a stable nitrosamine. The identified compounds are thus formed from secondary amines occurring as impurities in the solvent or being formed during the degradation reactions. As is shown in Figure 5, there are still some unidentified nitrosamines in the used solvent sample. These nitrosamines are formed from high molecular weight amines and have low volatility. Only in the first water wash stage low quantities of nitrosamines were found (see below).
The solvent specific nitramine (MEA-NO2) was detected at a concentration of approximately 2 mg/L (Table 3).
3.2 Analysis of wash water samples
MEA was periodically measured in the wash water from both water wash sections. The wash water sections are specifically designed to physically absorb gaseous and entrained aqueous MEA before the depleted flue gas is emitted to atmosphere. Figure 6 shows that the liquid phase concentration in the first wash water section (Lower wash water – right y-axis) was about 100 times higher than the upper section (Upper Water Wash – left y-axis). The results from 16/12-2013 show higher results, the temperature in the flue gas was 47°C and this will give higher MEA concentrations. Going from 30 to 40 wt% MEA in the solvent, will also give higher MEA concentrations in the water wash sections and this is measured at 19/2-2014, where the solvent MEA concentration was 40 wt%.
Methylamine and minor amounts of ethylamine were also found in water wash samples, as presented in Table 3.
Figure 6 clearly demonstrates the effectiveness of two water wash sections.
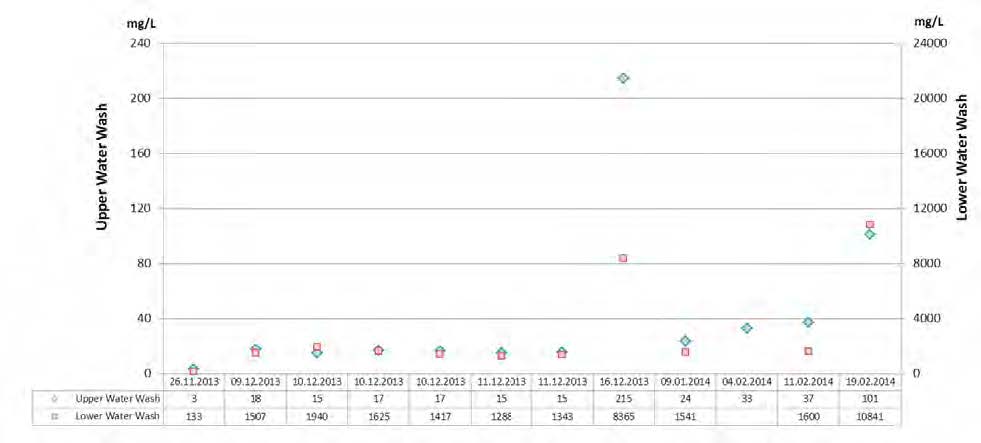
Figure 6: MEA concentrations in wash water 1 and 2.
The concentration of alkylamines, nitrosamines and nitramines in wash water samples are given in Tables 2 and 3. TONO were above detection limit only in the first water wash section, in one of two samples. This clearly indicates that nitrosamine volatility is low and that nitrosamines escaping from the solvent are efficiently captured in the first water wash section. No generic or solvent specific nitramines were found in either of the wash water sections.
Methylamine and minor amounts of ethylamine were found in low concentrations (μg/L) and it is seen that the water wash also has effect of these volatile compounds. No generic or solvent specific nitramines were found in either of the wash water sections.
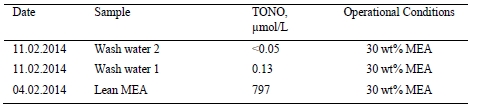
Table 2. TONO (Total nitrosamines) measurement, measured by Ramboll (flue gas, wash water) and Sintef (lean MEA).
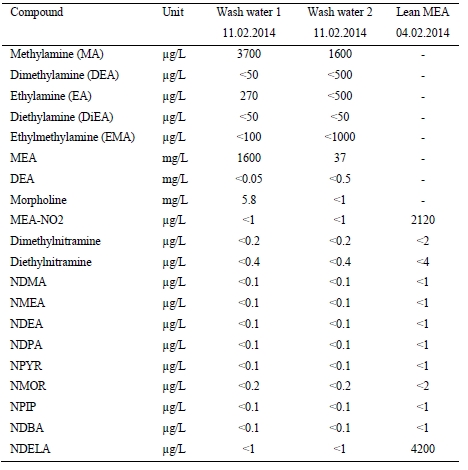
Table 3. Degradation components in solvent and wash water measured by Ramboll.
3.4 Analysis of gas emission samples
Thirteen manual isokinetic sampling emission campaigns were conducted during the MEA-campaign. All emission samples were collected by TCM DA, except one which was performed by FORCE Technology. The amine plant operating conditions and detailed emission results are given in Tables 4 to 6. All nitrosamine and nitramine emissions were below detection limits. Emissions of alkyl amines were limited and only methylamine is quantified in the low ppb range. Possible emission of unknown compounds has been investigated via PTR-TOF-MS. A list of identified or tentatively identified compounds is given in Table 7. No alkylamines, nitrosamines and nitramines were detected by PTR-TOF-MS.
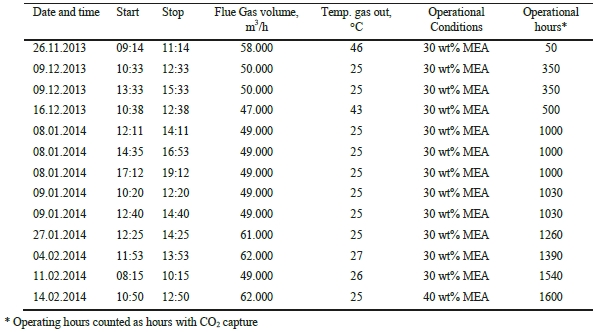
Table 4. List of emission measurements during the MEA campaign.
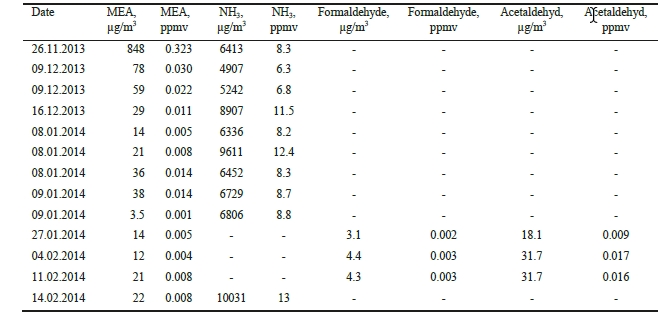
Table 5. Result from isokinetic gas emission measurements during the MEA campaign.
Figure 6 and Figure 7 display atmospheric emission results of MEA and NH3 from absorber outlet over the entire campaign. Figure 6 display emission results from the FTIR and PTR-TOF-MS analyzer in comparison with results from manual isokinetic sampling and analysis. The MEA FTIR results are not considered to be reliable in the low ppm range, since they are below/around the detection limit. The first measurement (performed on the 26th of November) showed emissions above 300 ppb. The reason for the higher amine emission in the first measurement is related to amine plant operating conditions. The NH3 emissions were reasonably low and as expected for MEA.
According to TCM DA experience the aldehyde concentrations were varying from low ppbv to several hundred ppbv during operations. Results found in this campaign and earlier campaigns are in agreement, and they are confirmed by third party. The FTIR is not measuring aldehydes below 1 ppm, but PTR TOF gives a good agreement to results found by isokinetic sampling and analysis, see tables 7 and 8. The PTR TOF is a good candidate for a reliable online analyzer of aldehydes in the ppb range.
Comparison of emission results from three sampling and analysis methods is somewhat tricky as there are some fundamental differences like; sampling point, sample extraction principles and sample transfer to the collecting or detection units. In this case manual samples are collected on the top of absorber using isokinetic extraction principles while the online methods are extracted non-isokinetic and switched in through a 101 meter long sampling line. Hence a comparison of MEA emission data can reflect differences in the sampling configuration. The analytical differences are first of all related to instrumental detection limits. Taking sampling and analytical differences into account the comparison of results is summarized and illustrated in figure 6. It is clear that the FTIR data is affected by high detection limit and by then increased uncertainty for this low ppm to ppb-level. Results from manual sampling and online PTR-TOF-MS are first of all according to both methods reported in a low concentration level (0,001 – 0,3 ppmv). The variation between the two data sets is significant and in general manual sampling reflects lower values than online PTR-TOF-MS results. Based on TCM-experience it is likely that the different sampling set-up explain this. TCM has experienced during this MEA campaign, that switch between different sampling points and long heated sampling lines are challenging and need to be tested more and further optimized to secure stable and representative gas composition.
The manual isokinetic sampling and analysis is considered to be a reference method for TCM DA. Isokinetic
sampling and analysis is verified by two independent third party companies (during earlier campaigns by Kema/SGS and FORCE, and in this MEA campaign by FORCE).
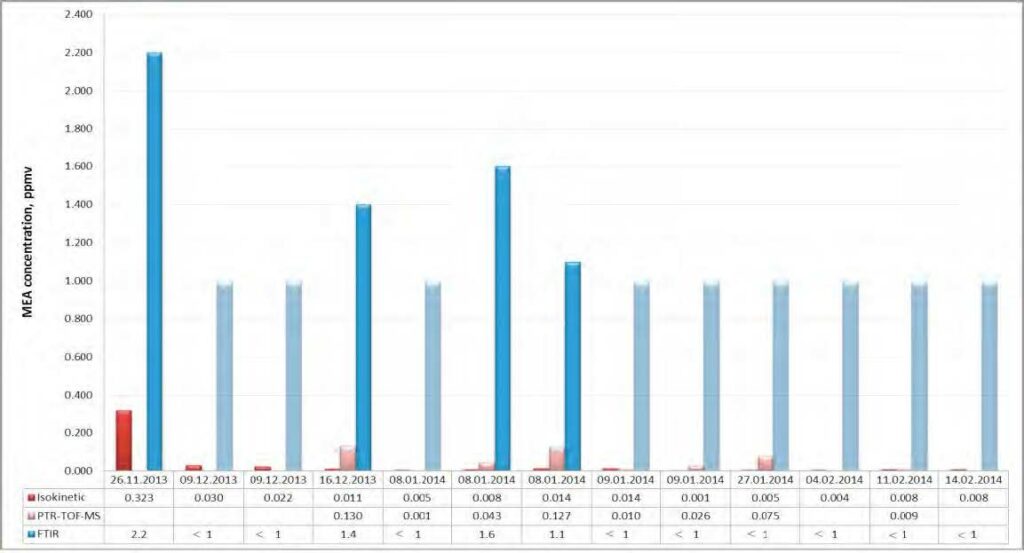
Figure 6: MEA emissions determined by different analyzer techniques during the campaign. Results on the FTIR below detection limit (<1 ppmv) are colored lighter blue.
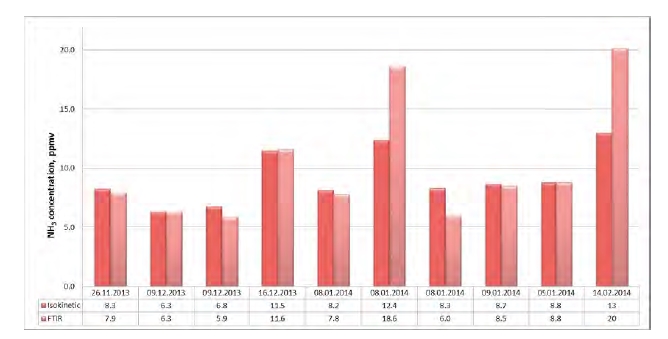
Figure 7: NH3 emissions determined by different analyzer techniques during the campaign Table 6. Degradation components in Flue gas out of absorber from isokinetic gas emission measurements.
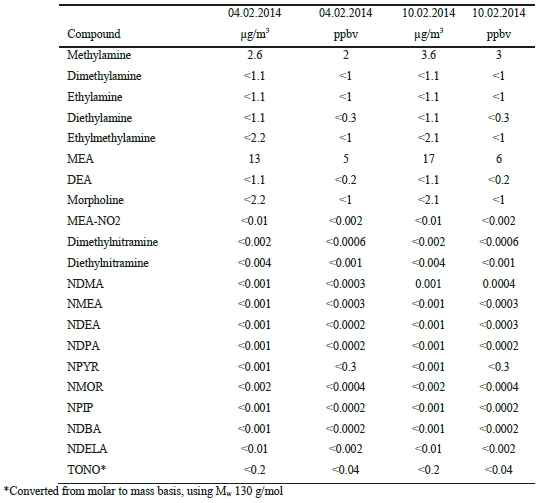
Table 6. Degradation components in Flue gas out of absorber from isokinetic gas emission measurements.
PTR-TOF-MS was also used for screening of potential other emissions. A list of identified or tentatively identified compounds is given in Table 7. It is noted that PTR-TOF-MS did not detect any emissions of alkylamines, nitrosamines or nitramines.
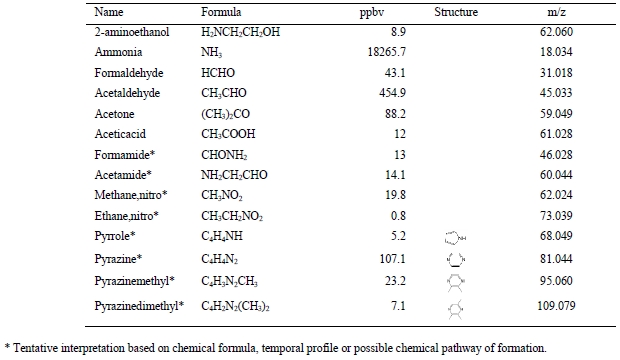
Table 7. Results from PTR-TOF-MS measurements on 11.02.2014, 08:15-10:15. Estimated uncertainty in measurements is +20%.
3.4 Third party gas emission measurement
One third-party emission measurement was done on January 6. FORCE Technology carried out isokinetic sampling onto a solid sorbent (Thermosorb/N) in combination with condensate collection in an impinger. The condensate was analyzed separately. Analysis of collected samples was done by Isconlab GmbH. The results show that all nitrosamine and nitramine emissions were below detection limits.
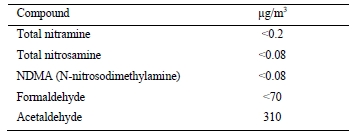
Table 8. Results from third part measurement on 6th of January, done by FORCE Technology.
4. Conclusion
Extensive atmospheric emission monitoring has been conducted at the CO2 Technology Centre Mongstad (TCM DA) during amine based post-combustion CO2 capture. The TCM DA amine plant was operated with the aqueous MEA solvent system treating flue gas from a combined heat and power plant (CHP). Emission monitoring was conducted by a Fourier Transform Infrared (FTIR) Spectrometry analyzer, a Proton Transfer Reaction Time-of- Flight Mass Spectrometry (PTR-TOF-MS) analyzer, and manual isokinetic sampling followed by off-line analysis in the laboratory.
Atmospheric emissions of monoethanolamine (MEA) were very low throughout the complete campaign, and determined to be in the parts per billion (ppb) range. Atmospheric emissions of MEA amine based degradation products such as nitrosamines and nitramines were below detectable levels. Atmospheric emissions of ammonia (NH3) were in the low ppm range, and alkyl amines in the low ppb range.
Absorber wash water sections were found to effectively reduce possible atmospheric emissions from amine based solvent system.
Acknowledgements
The authors gratefully acknowledge the staff of TCM DA, Gassnova, Statoil, Shell, Sasol, and Aker Solutions for their contribution and work at the TCM DA facility, and the staff of the Statoil CP laboratory for their contribution and work with the LC-MS measurements.
The authors also gratefully acknowledge Gassnova, Statoil, Shell, and Sasol as the owners of TCM DA and Aker Solutions for their financial support and contributions.
Appendix A. Abbreviations
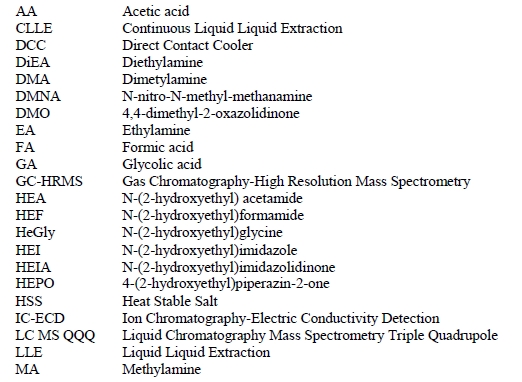
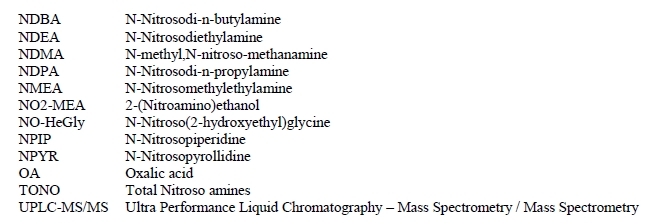
References
- Thimsen D, Maxson A, Smith V, Cents T, Falk-Pedersen O, Gorset O, Hamborg E S. Results from MEA testing at the CO2 Technology Centre Mongstad. Part I: Post-Combustion CO2 capture testing methodology. Energy Procedia; 2014.
- Hamborg E S, Smith V, Cents T, Brigman N, Falk-Pedersen O, De Cazenove T, Chhaganlal M, Feste J K, Ullestad Ø, Ulvatn H, Gorset O, Askestad I, Gram L K, Fostås B F, Shah M I, Maxson A, Thimsen D. Results from MEA testing at the CO2 Technology Centre Mongstad. Part II: Verification of baseline results. Energy Procedia; 2014
- Brigman N, Shah M I, Falk-Pedersen O, Cents T, Smith V, De Cazenove T, Morken A K, Hvidsten O A, Chhaganlal M, Feste J K, Lombardo G, Bade O M, Knudsen J, Subramoney S C, Fostås B F, De Koeijer G, Hamborg E S. Results of amine plant operations from 30 wt% and 40 wt% aqueous MEA testing at the CO2 Technology Centre Mongstad. Energy Procedia; 2014.
- Eirik Falck da Silva, Karl Anders Hoff, Andy Booth; Energy Procedia 37 (2013) 784-790
- Gelein de Koeijer, Vibeke Randgaard Talstad, Sissel Nepstad, Dag Tønnesen, Olav Falk-Pedersen, Yolandi Maree, Claus Nielsen; International Journal of Greenhouse Gas Control 18 (2013) 200-207
- In: Norwegian Climate and Pollution Agency (Ed.), Tilllatelse til virksomhet etter forurensningsloven for TCM DA (Norwegian only), Norwegian Climate and Pollution Agency, Oslo, Norway (Now: Norwegian Environmental Agency (Miljødirektoratet))
- http://www.gassnova.no/en/ccs-projects/full-scale-mongstad/results-from-the-second-round-of-studies-of-the-health-and-environmental-issues-of-amines
- Toine Cents, Øyvind Ullestad, Anne Kolstad Morken, Olav Falk-Pedersen, Otto Bade, Gunnar Schade, Liang Zhu, Claus Nielsen. Measurement of emissions from the amine based CO2 capture plant at CO2 Technology Centre Mongstad. TWELFTH ANNUAL CONFERENCE ON CARBON CAPTURE, UTILIZATION AND SEQUESTRATION May 13 – 16, 2013 • David L. Lawrence Convention Center • Pittsburgh, Pennsylvania
- Jan Mertens, Marie-Laure Thielens, Jacob Knudsen and Jimmy Andersen. Monitoring and impacting gaseous emissions in post combustion carbon capture. IEAGHG PCCC1, 1st Post Combustion Capture Conference
- Jan Mertens, Dominique Desagher, Marie-Laure Thielens, Han Huynh Thi Ngoc, Helene Lepaumier, Purvil Khakaria and Earl Goetheer. On- and off-line ethanolamine and ammonia emission monitoring in PCCC. IEAGHG PCCC2, 2nd Post Combustion Capture Conference.
- Zhu, L., Schade, G. W. & Nielsen , C. J. Real time monitoring of emissions from monoethanolaminebased industrial scale carbon capture facilities. Environmental Science & Technology 47, 14306-14314 (2013)
- Zhu, L., Schade, G. W. & Nielsen , C. J. TCM emission measurements: results from PTR-ToF-MS measurements during use of MEA 2012. (2013).
- Aslak Einbu, Eirik DaSilva, Geir Haugen, Andreas Grimstvedt, Kristin Giske Lauritsen, Kolbjørn Zahlsen, Terje Vassbotn, Energy Procedia 37 (2013) 717-726.
Health risk analysis for emissions to air from CO2 Technology Centre Mongstad (2013)
Gelein de Koeijera,∗, Vibeke Randgaard Talstada, Sissel Nepstada, Dag Tønnessenb, Olav Falk-Pedersena, Yolandi Mareea, Claus Nielsenc
a TCM DA/Statoil, NO-5494 Mongstad, Norway b NILU, NO-2027 Kjeller, Norway c University of Oslo, NO-0361 Oslo, Norway ∗ Corresponding author
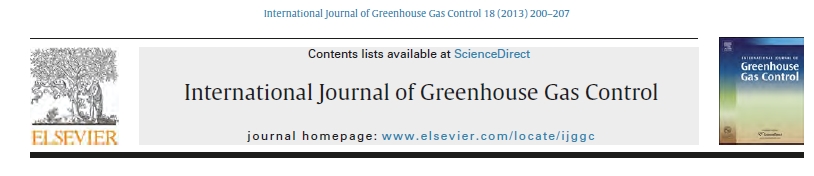
Abstract
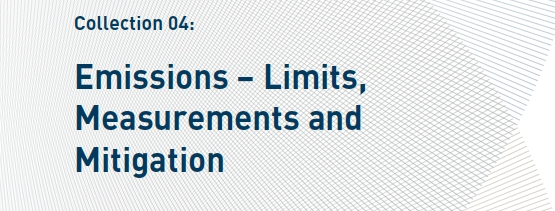
A health risk analysis for the emissions to air from the CO2 Technology Centre Mongstad (TCM) has been executed. TCM is the world’s largest facility for testing and improving technologies for CO2 capture, and is located at the West coast of Norway. The risk analysis was an important fundament for the application for an emission permit for the amine based post-combustion CO2 capture unit. The highest risk was assessed to be the exposure of the population to uncertain concentrations of nitrosamines and nitramines in air and drinking water. Nitrosamines and nitramines are groups of possible degradation products formed from amines. The components within these two groups have variable degrees of carcinogenicity. Nitrosamines are formed from amines in the CO2 capture process and in the atmosphere, while nitramines are assumed to form only in the atmosphere. The risk was analyzed by comparing the sum of concentrations of nitrosamines and nitramines in air and fresh water with recently available guidelines. The concentrations were obtained by modelling atmospheric chemistry, dispersion, deposition by precipitation and degradation in fresh water with novel methods that were developed during the application process. Moreover, the nitrosamine and nitramine concentrations were measured in air and fresh water lakes prior to start-up as a baseline. TCM’s conclusion was that the risk was acceptable. The Norwegian Climate and Pollution Agency granted TCM a permit in November 2011.
This article is behind a paywall. Futher information: https://www.sciencedirect.com/science/article/abs/pii/S1750583613002788?via%3Dihub
Real-Time Monitoring of Emissions from Monoethanolamine-Based Industrial Scale Carbon Capture Facilities (2013)
Liang Zhu,† Gunnar Wolfgang Schade,†,‡ and Claus Jørgen Nielsen*,†
†Department of Chemistry, University of Oslo, P.O. Box 1033 Blindern, 0315, Oslo, Norway
*Supporting Information

Abstract
We demonstrate the capabilities and properties of using Proton Transfer Reaction time-of-flight mass spectrometry
(PTR-ToF-MS) to real-time monitor gaseous emissions from industrial scale amine-based carbon capture processes. The benchmark monoethanolamine (MEA) was used as an example of amines needing to be monitored from carbon capture facilities, and to describe how the measurements may be influenced by potentially
interfering species in CO2 absorber stack discharges. On the basis of known or expected emission compositions, we investigated the PTRToF-MS MEA response as a function of sample flow humidity, ammonia, and CO2 abundances, and show that all can exhibit interferences, thus making accurate amine measurements difficult.
This warrants a proper sample pretreatment, and we show an example using a dilution with bottled zero air of 1:20 to 1:10 to monitor stack gas concentrations at the CO2 Technology Center Mongstad (TCM), Norway. Observed emissions included many expected chemical species, dominantly ammonia and acetaldehyde, but also two new species previously not reported but emitted in significant quantities. With respect to concerns regarding amine emissions, we show that accurate amine quantifications in the presence of water vapor, ammonia, and CO2 become feasible after proper sample dilution, thus making PTR-ToF-MS a viable technique to monitor future carbon capture facility emissions, without conventional laborious sample pretreatment.
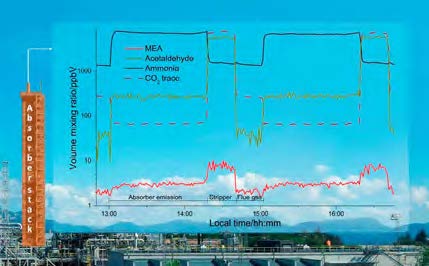
This article is behind a paywall. Futher information: https://pubs.acs.org/doi/10.1021/es4035045
Establishment of Knowledge base for Emission Regulation for the CO2 Technology Centre Mongstad (2013)
Yolandi Maree*a, Sissel Nepstada, Gelein de Koeijerb
aTCM DA, 71 Mongstad, 5954, Norway bStatoil ASA, Ark. Ebbellsvei 10, 7005 Trondheim, Norway *Corresponding author
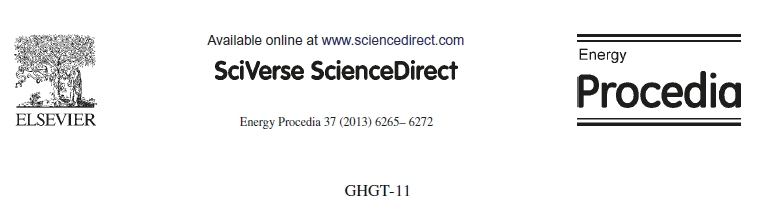
Abstract
The CO2 Technology Centre Mongstad (TCM) is currently regarded as the world’s largest CO2 capture technology test centre at 100 000 ton/year of CO2 capture capacity. The main aim of the project was to play an important role in the establishment of proven and cost efficient CCS (CO2 capture and storage) value chains. At first two technologies are tested, namely and amine plant (designed by Aker Clean Carbon) and a chilled ammonia plant (designed by Alstom) which is fed with two different flue gas sources. The latter will, by normal composition as well as CO2 recycle design, allow for a large band of CO2 concentrations available to the various technologies. In principle, the two flue gas sources along with the recycle steam will allow to simulate flue gasses from both gas- as well as coal fired applications.
The work presented here aims to discuss and introduce the interaction between the Norwegian Climate- and Pollution Agency (Klif) and TCM. The importance of these activities are highlighted by the challenges faced to ensure safe emissions levels in order to allocate the emissions and discharge permit and subsequent regulatory measures associated with this permit. Large uncertainties regarding worst case assumptions for emissions from the TCM amine plant had to be addressed in order to deduce safe levels for amine degradation products, like nitrosamine and nitramines. The latter are known carcinogens with variable carcinogenic properties. Thus, a two-folded approach was taken by firstly addressing the knowledge gap towards amine degradation products and their respective impact on health and environment. Secondly, close cooperation with Klif was required to establish relevant regulation for the CO2 capture facility.
In November 2011, an emission and discharge permit for TCM was approved by Klif. Regulatory levels for amines, ammonia, aldehydes and other flue-gas related species were depicted in the permit, both on immediate concentration levels as well as permitted annual levels and wider environment deposition concentrations. The paper concludes by looking at the first set of measured emissions parameters from the amine plant at TCM and considers the results in relation with the initial risk assessments associated with the permit.
1. Introduction
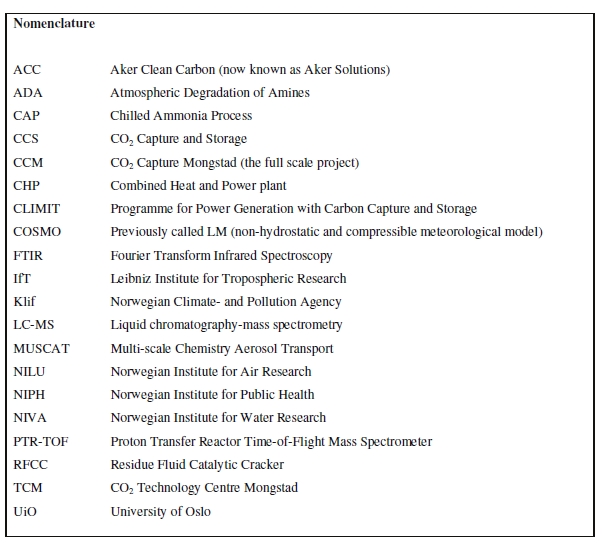
CO2 Technology Centre Mongstad (TCM), situated in Norway, currently stands as the largest facility for testing and improving CO2 capture technologies. TCM aims of playing an important role in establishing proven and cost efficient CCS value chains. Hence, the activities at TCM are focussed on progressing technology development by testing and improving CO2 capture technologies.
TCM is a company owned by Gassnova (the Norwegian state), Statoil, Norske Shell and Sasol. Initially two technologies will be tested, namely: an amine plant and a chilled ammonia plant. These two technologies will both be tested on two separate flue gas sources. The first source is off-gas from the residue fluid catalytic cracker (RFCC) at the Mongstad Refinery, and the second will be exhaust gas originating from the combined heat and power plant (CHP) at Mongstad. The two plants are designed to jointly capture ca 100 000 tons per year of CO2. The work presented here aims to introduce and discuss the interaction between the Norwegian Climate- and Pollution Agency (Klif) and TCM in the challenging period when safe emissions levels from TCM were being established and an emissions discharge permit for the TCM facility was thereafter granted by Klif. This paper continues the discussion on emissions raised in an earlier paper on TCM [1].
2. Emissions and discharge permit application
TCM applied for an emissions and discharge permit from Klif in September 2010. At the time of submission of the application, large uncertainties existed for the worst case scenario for emissions from the TCM amine plant. The highest level of uncertainty was related to the possible impacts that amine degradation products, like nitrosamine and nitramines, could have on the public health in close vicinity (<50 km) from the facility.
Nitrosamine and nitramines are partly formed through the degradation of amines within the process itself and partly through the atmospheric reaction involving OH- radicals. Both of these component groups may consist of a wide range of species dependant on the mother amine and the reaction conditions, and some of their resulting nitrosamines and nitramines are known to have carcinogenic effects. For this reason, TCM placed a lot of emphasis on establishing a transparent risk analysis, which includes allowable risk limits relevant to these components. The knowledge base on the toxicology of nitramines is even less than for nitrosamines, but literature and studies to date indicate that nitramines are less carcinogenic than nitrosamines [3].
Focus at TCM during the year following the submission of the permit was on reducing the technical knowledge gap of these degradation components, while the regulating authorities in parallel tasked themselves to establish regulation and limitations levels. The technical knowledge gap was addressed by close interaction with programs like CLIMIT as well as the CCM project, dually by considering and defining the formation of nitrosamine and nitramine species during day and night operation as well as describing the exposure effect of these components to the immediate and wider areas around the TCM facility. The impact and risk of these components were investigated based on both human exposures to air, as well as possible negative effects by potential changes in drinking water quality.
A large number of acknowledged research facilities and institutes were engaged by TCM to address and assess various parts in the amine degradation products knowledge gap. The findings of the various studies have been/will be published by the different institutions as well as by Klif [2]. Therefore this paper will not reiterate these results, but rather describe the events and specific interaction that took place between the regulator (Klif) and TCM following the submission of the emissions and discharge permit application in September 2010. The application, supporting documentation and the resulting permit describe the first risk analysis from amine emissions. De Koeijer et al [11] presents the results on which the health risk analysis was based in greater detail. The main intention is experience transfer to other CO2-testing and full scale endeavours that in the future are applying for an emission and discharge permit and/or other regulation activities.
2.1 Initial amine degradation product knowledge base
The initial permit application was lodged while acknowledging that a large knowledge gap existed in terms of the impact of amine slip to air and the consequences of this to the direct and indirect environment. The standing knowledge base at that time was the intermediate results from the ADA (atmospheric degradation of amines) research campaign headed by the University of Oslo [4]. The latter was focused at identifying the gas phase photochemical degradation products of amine in the atmosphere, quantifying the products from photo-oxidation of amines, verification and updating of existing atmospheric photo-oxidation schemes as well as assessing aerosol formation during gas phase degradation of amines in air. In parallel to the ADA campaign, preliminary dispersion models developed by NILU indicated that significant levels of amine degradation products may be deposited close within the vicinity of the TCM process boundaries [5].
Subsequently, the knowledge at the time directed towards possible health impacts of amines and their degradation products, but no specified guideline values for nitrosamines and nitramines existed. The Norwegian Institute for Public Health (NIPH) was therefore approached to assess and recommend regulatory values for nitrosamines and nitramines as a first step towards discharge permissions for the TCM facility.
2.2 Studies aimed at closing the knowledge gap
An all encompassing research campaign was launched in order to address the prevailing knowledge gap associated with possible emissions from TCM. Three major areas were targeted: firstly, the atmospheric chemistry and dispersion of amines and their degradation products. Secondly, the fate of nitrosamines in water by means of biodegradation was investigated and the final activity was to establish baseline assessments in air, water and soil. During the selection process emphasis was placed in utilising well established and recognised institutions for these studies.
Main findings from the campaigns indicated that nitrosamine and nitramines are partly formed through the degradation of amines within the process itself and partly through the atmospheric reaction involving OH- radicals [4]. Formation of nitrosamines and nitramine species are highly dependent on the mother amine and reaction conditions. The various nitrosamine and nitramine component groups are known to vary in carcinogenic properties and thus a comprehensive dispersion model was compiled in order to assess the worst case and likely case concentrations for these species in ambient air and deposition in fresh water [11]. Emphases during the studies were placed on reducing the uncertainty while re-assessing assumptions made in the first dispersion modelling work. Furthermore, investigations were also launched into possible emissions levels of nitrosamines and nitramines species from other industrial plants and deducing whether or not these components are being regulated elsewhere [12].
In March 2011 the NIPH published guideline values for nitrosamine and nitramine species by stating that these component groups must not exceed 0.3 ng/m3 for air concentrations and 4 ng/l for fresh water sources or drinking water when considering a 1 in 10-6 cancer risk for lifetime exposure [2]. Initial “worst case” assumptions for the dispersion model indicated that these guideline values will not be exceeded and thus more emphasis was placed in refining the atmospheric chemistry assumptions while introducing possible environmental degradation of these species through biodegradation studies. The purpose of the “worst case” study was to include worst estimates on the different aspects of formation and transformation, while a more likely parameter update was launched in order to include new findings and thus calculating the most likely impact involving air and water quality. The latter was further referred to as the “likely case”, which in turn resulted in the following main conclusions [6]:
- Air concentrations of nitrosamines and nitramines (as a sum) are expected to be lower by a ratio of 3 to 5 when compared to the NIPH guidelines.
- Water concentrations of nitrosamine and nitramines (as a sum) are expected to be lower by a ratio of 16 to 22 when compared to the NIPH guidelines.
The likely case dispersion model included findings from other parallel studies by considering the reduction of nitrosamines in water by means of photolysis (3 weeks life-time) and possibly biodegradation (half-life of 40 to 400 days) [7]. Biodegradation reduction of nitramines of 33% over 28 days was also included [8].
Baseline assessments showed detectable amounts of neither nitrosamines nor nitramines [9]. The baseline study paired with the theoretical deposition of nitrosamine and nitramines in air and water therefore strengthened the position that the activities of TCM will not exceed the NIPH guidelines and is thus unlikely to be responsible for any detrimental health or environmental effects.
3. Updated information to Klif and public participation
All updated information was presented to Klif during the first and second quarters of 2011, including the final reports to all of the studies launched since the original emissions and discharge permit submission. During the processing time of the information by Klif, TCM embarked on a community information program in which several meetings were held with various community stakeholders as well inviting any interested parties to the TCM site. It was the first emissions and discharge permit of its kind and therefore public understanding of the risks and implications was deemed crucial if the application was to be successful – not only for the operation at TCM, but it was clear that the way was being paved in which future CCS projects will be governed in Norway and possibly the most of Europe. An open public hearing was also held in the second quarter of 2011 in which both Klif and municipal representatives participated, along with nearly 50 other interested parties. This, along with close communication with the Lindås and Austrheim municipalities, a general understanding towards the risks and acceptance towards the mitigation activities by TCM was received.
4. Final permit approval and conditions
More than a year after the initial application, the final emissions and discharge permit was allocated to TCM in November 2011, with the regulatory levels relevant to air emissions presented in Table 1.
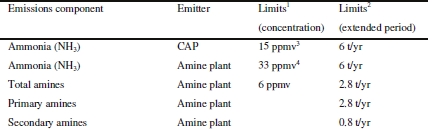
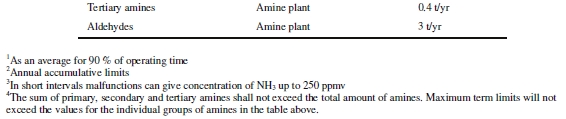
Table 1. Regulatory levels for air emissions according to the TCM emissions and discharge permit [2].
The permission as shown in Table 1 is independent of solvent used. In order to obtain this independence in solvent, the amines were grouped in primary, secondary and tertiary amines based on the risk of each group towards nitrosamines and nitramines formation. The variability of these groups within different solvent compositions will require new evaluations and risk assessments before being introduced to the TCM plant [2].
Further to direct air emissions at source, restrictions are imposed on concentration levels calculated for fresh water and dispersed air for nitrosamines and nitramines. The permit states that emissions from TCM shall not lead to that the calculated concentration of the sum of nitrosamines and nitramines exceed
0.3 ng/m3 for air concentrations and 4 ng/l for fresh water sources or drinking water [2]. These guidelines were derived from direct recommendations as published by the NIPH [3].
Apart from air regulations, the emissions and discharge permit covers all areas of environmental concern. This includes liquid discharges, solid wastes, noise and others. All of the individual regulated areas will not be discussed here as it is regarded as well known areas and have been established by the various governing authorities world-wide.
5. Ongoing and updating studies
In an effort to continuously update the knowledge base at TCM, which in turn will benefit future full scale projects, some study areas have been selected to be updated past the allocation of the permit. The first is an updated dispersion model conducted with IfT (Leibniz Institute for Tropospheric Research) and the other is the biodegradation of nitramines.
The updated dispersion model that is being developed by the team at IfT is aimed at providing the following improvements to the current model:
- The model will be based on COSMO-MUSCAT and thus improved meteorology will be used in the base model [10].
- The model will include the atmospheric chemistry of amines and thus present the true theoretical yields of amine degradation products to the wider environment.
- The model will provide a more detailed tool which will enable the evaluation of other solvents and will not be restricted to MEA (mono-ethanol amine) solvents.
The theoretical yields of nitrosamine and nitramines in the ADA study showed that more nitramines than nitrosamines will possibly be formed [4]. And prior biodegradation studies also indicated that nitramines are more readily biodegradable then their nitrosamine counterparts [8]. These two indications therefore led to the establishment of biodegradation studies with SINTEF on ethanolnitramine (MEA-NO2), dimethylnitramine (DMNA), N-nitropiperazine (PZ-NO2), methylnitramine (MNA), 2-methyl-2- (nitroamino)-1-propanol (AMP-NO2), diethylnitramine (DENA) and methylethylnitramine (MENA). The selection of these components are believed to cover a broad range of future solvents that may be tested at TCM. The results from these studies will in turn also be included in the updated dispersion model developed by IfT.
6. Environmental follow up program, latest results and conclusion
The TCM emissions and discharge permit, as outlined by Klif, entails an extensive environmental follow- up and monitoring plan. Monthly and annual reports have to be compiled in which all discharges and possible environmental impacts are clearly identified and quantified. The main aspects of the ongoing environmental follow-up and monitoring plan are:
- Terrestrial and fresh water surveys close to the TCM boundary fence. A background survey was completed by NIVA and bi-annual surveillance will be conducted during operation [13].
- Noise modelling and surveillance (these activities are done in conjunction with the refinery at Mongstad).
- A comprehensive air monitoring program will be followed in which air quality will be measured with online instruments (FTIR), third party measurements (PTR-TOF-MS with UiO) as well as manual isokinetic sampling conducted in-house (analysed through LC-MS).
During July 2012 the amine plant was started up, which allowed for initial environmental monitoring during August 2012. Considering the fact that this was the first month of operation on MEA solvent, very promising results were gathered with respects to amine emissions. Firstly, very low levels of amines were detected at the bottom of the 101m sample line by the on-line FTIR (less than 1ppm) as well as the PTR-TOF-MS (less than 100 ppb). The FTIR set-up typically allows for accuracy of 1ppm and is thus in line with the much more accurate PTR-TOF-MS which is connected to the same sample line as the FTIR. Secondly, the isokinetic sampling campaigns at the absorber exhaust have shown to exhibit the same low levels of MEA once analysed through LC-MS (less than 0.1ppm). At this early stage the first results serve as a confirmation that the initial risk assessments for TCM DA are realistic and translates that the NIPH levels are not likely to be exceeded for this solvent. It is important to note, however, that these results are not representative enough to be conclusive due to process variation, but it serves as a very promising first indication.
The main ambitions for TCM at conception were to test, verify and demonstrate CO2 capture technologies while reducing costs, technical, environmental and financial risks. The ground made in terms of establishing the emissions and discharge permit with the Norwegian regulator was the first step in achieving these ambitions in environmental terms. The methodology, interaction and end result gained here will more than likely set the trend for new projects of similar stance throughout Europe and the rest of the world. Other than breaking ground in terms of regulation, the activities resulting from the work presented here also achieved an increase in global understanding in amine chemistry, amine degradation products and their respective environmental impact which is not restricted to CCS based projects alone.
Acknowledgements
This work was funded by the owners of TCM DA (Gassnova, Statoil, Shell and Sasol) in support of their application for an emission and discharge permit for TCM. Aker Clean Carbon is thanked for their contribution to the emission profiles.
References
- De Koeijer G, Enge Y, Sanden K, Graff OF, Falk-Pedersen O, Amundsen T, Overå S, CO2 Technology Centre Mongstad. Design, functionality and emissions of the amine plant. Energy Procedia 2011; 4:1207-1213.
- Climate and Pollution Agency. Reports supporting TCM’s emission permit application. Website http://www.klif.no/nyheter/brev/tcm_tillatelse171011.pdf . Published 2011.
- Låg, M., Lindeman, B., Instanes, C., Burnborg, G., Schewarze, P. Norwegian Institute of Public Health. Health effects of amines and their derivatives associated with CO2 capture. Published 29 March 2011, Folkehelseinstituttet http://www.fhi.no.
- Norsk Institutt for Luftforskning (NILU). Atmospheric degradation of Amines (ADA). Report OR 2/2011. Published http://www.klif.no/Aktuelt/Horinger-og-kunngjoringer/2010/2010-1038/?tab=3.
- Norsk Institutt for Luftforskning (NILU). CO2 Technology Centre Mongstad – updated air dispersion calculations. Report OR41/2010. Update of OR 12/2008. Published http://www.klif.no/Aktuelt/Horinger-og-kunngjoringer/2010/2010- 1038/?tab=3.
- Norsk Institutt for Luftforskning (NILU). Update and improvement of dispersion calculations for emissions to air from TCMs amine plant. Part II-Likely case nitrosamines, nitramines and formaldehyde. Report 52/2011. Published http://www.klif.no/Aktuelt/Horinger-og-kunngjoringer/2010/2010-1038/?tab=3.
- Brakstad, O.G., Booth, A., Zahlsen, K (SINTEF). Biodegradation of nitrosamines in water. SINTEF A18258. Published http://www.klif.no/Aktuelt/Horinger-og-kunngjoringer/2010/2010-1038/?tab=3.
- Dye, C., Fjellsbø, L. M. B., Dusinska, M. (NILU). Nitramine analysis procedures development and screening toxicity study. Report 15/2011. Published http://www.klif.no/Aktuelt/Horinger-og-kunngjoringer/2010/2010-1038/?tab=3.
- Tønnesen, D., Dye, C., Bøhler, T. (NILU). Baseline study on air and precipitation quality for CO2 Technology Centre Mongstad. Report OR 73/2011. Published http://www.tcmda.com/en/Technology/Environmental-studies.
- Wolke, R., Knoth, O., Hellmuth, O., Schröder, Renner, E. The parallel model system LM-MUSCAT for chemistry- transport simulations: Coupling scheme, parallelization and application. In Joubert, G. R., Nagel, W.E., Peters, F.J., Walters, W.V., Eds. Parallel computing: Software technology, algorithms, architectures and applications. Elsevier 2004; 363-370.
- De Koeijer, G., Talstad, V. R., Nepstad, S., Tønnessen, D., Nielsen, C. Health risk analysis for emissions to air from CO2 Technology Centre Mongstad. Pending article, 16 pages.
- Selin, N. E., Environmental guidelines and regulations for nitrosamines: A policy study. Prepared for CO2 Technology Centre Mongstad by Massachusetts Institute of Technology (MIT), department of earth, atmospheric and planetary sciencies. July 2011, 21 Pages.
- NIVA. Terrestrial and aquatic baseline study and monitoring programme for CO2 Technology Centre Mongstad. Report for TCM DA by NIVA, report sno 6311-2012, 97 pages.
Health and environmental impact of amine based post combustion CO2 capture (2013)
Erik Gjernesa*, Laila Iren Helgesena, Yolandi Mareeb
aGassnova SF, Dokkvegen 10, NO-3920 Porsgrunn, Norway bTCM DA, 71 Mongstad, NO-5954, Norway
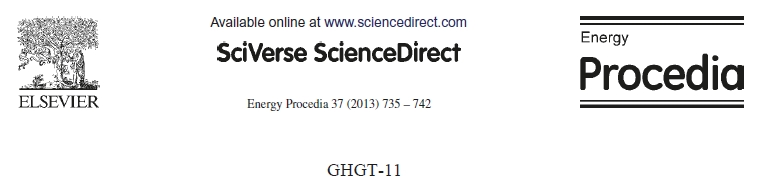
Selection and/or peer-review under responsibility of GHGT
doi: 10.1016/j.egypro.2013.05.162
Abstract
A post-combustion amine based CO2 capture plant is associated with minor release of amine and amine degradation products to the atmosphere along with the treated flue gas. The possible health and environmental effect of this have been investigated extensively through the Norwegian CLIMIT-program, the CO2 Technology Centre Mongstad (TCM) and the planning of a full scale capture plant at Mongstad (CCM). Based on flue gas from a combined cycle gas power plant, CCM are 25 000 and 1 000 000 t/yr CO2, respectively. Special attention has been given to nitrosamines and nitramines and the investigations have provided new knowledge on their formation, degradation and dispersion, going from the capture plant towards the end point in the nature. The initiatives and programmes have also included means of emission reduction as well as refining of measurements techniques. Computer models for dispersion calculation and evaluation of maximum level of components in air and drinking water are important and are part of the environmental permit for TCM. Up to now, this new knowledge has significantly reduced the risk perception for the release of amine and amine degradation products to the atmosphere.
1. Introduction
The possible health and environmental impact of amines and amine degradation products being released to the atmosphere has received a lot of attention in Norway and a substantial amount of research has been carried out. The majority of the investigations has been financed from the Norwegian CLIMIT- program (co-founded with industry) and through the planning of the amine based CO2 capture plants at the CO2 Technology Centre Mongstad (TCM) and the full scale plant at Mongstad (CCM). These programs and initiatives are all sponsored by the Norwegian state. This comprehensive work has provided a better understanding of the various issues of amine release to the atmosphere and up to now this new knowledge has significantly reduced the risk perception.
The investigations have been initiated in order to obtain a knowledge base enabling a risk assessment of environment and human exposure to amines and their possible degradation products. The studies include chemistry and degradation of amines in the capture plant, emission to air and emission reduction technologies, atmospheric degradation of amines and environmental fate studies. Thus the whole chain from the capture plant to the final end point is covered. Up to now this has resulted in 55 projects executed by international as well as Norwegian institutes. The total budget exceeds 20 million euro, and the programs are in progress. The CCM activities are part of the qualification of amine technology and have the largest budget, followed by the CLIMIT-program and TCM. CLIMIT is supporting RD&D for CO2 capture, transport and storage, and has since 2008 also built up a special research portfolio on impact of amine use application for a discharge permit. Impact of amine uses has also been investigated by others, but the current Norwegian initiatives are the largest and most comprehensive.
2. Investigations
An assessment from 2008 [1], based on available literature data, indicated that amine emission from capturing 1 million tons of CO2 per year from a combined cycle gas power plant could imply a health and environmental risk. One important observation was that relevant experimental data was scarce. The possible routes of atmospheric degradation of emitted amine into nitrosamines and nitramines is an important part of an assessment, and by 2012 there are strong evidences that all major knowledge gaps in the relevant atmospheric chemistry are closed. This is the result of a focused effort on atmospheric chemistry through a series of comprehensive experimental- and theoretical investigations of amines and their degradation products. The amine chemistry in the capture plant itself has also been investigated and the degradation routes and conditions for provoking formation of substances such as nitrosamines are found. This knowledge is part of the activities for qualifying amines for CCM at Mongstad. Means of reducing emission from the absorber to the atmosphere are developed and tested. The stability of the nitrosamines and nitramines in the environment is an additional important parameter. Experimental investigations show that nitrosamines decompose more easily than nitramines in the environment and this is important for assessing the final concentration in air and drinking water. Overview of the activities can be found elsewhere [2,3,4].
2.1 Capture plant process
In an amine based capture plant the specific amine used as well as operational conditions determine candidates for emission to air. Flue gas degradation of monoethanolamine (MEA) as a function of O2, NOX and temperature has been studied by Fostås et al [5]. They used a laboratory sized absorber column in combination with post treatment of solvent in an autoclave and found the amine diethanolamine (DEA) and the corresponding nitrosamine, nitrosodiethanolamine (NDLA), as well as traces of nitrosodimethylamine (NDMA), nitrosomorpholine (NMOR) and methylamine (MMA). Their methodology has been brought forward in the technology qualification amine program for CCM.
Each of the participating vendors in the qualification program for CCM will get access to a lab rig in order to test process degradation of amines according to a test protocol which have been developed by Sintef [6]. The lab rig is a compact portable unit simulating the absorber/desorber process of a normal CO2 capture plant. The solvent is in this rig tested through extra high level of O2 and NOX in the exhaust gas and with extra high temperature in the desorber column. During the first test run by Sintef using MEA, nitrosamines were as expected, detected. By the end of 2012 four technology vendors, Mitsubishi Heavy Industries, LTD., Siemens AG, Aker Clean Carbon and Huaneng-CERI Powerspan Joint Venture will have completed this phase of the qualification program.
It is foreseen that further measures to reduce emissions from amine capture plants may be required. Amines and degradation products are expected to be found both in the gas phase and in droplet carry over from the absorber. Thus emission reduction technologies applicable for removing both gaseous components and droplets are of interest. The following technologies were ranked according to applicability and maturity [7]:
- An extra water wash section on top of the absorber
- High efficiency demisters and filters
- Acid wash in the final washing section on the absorber top
- UV treatment of lean amine, wash water or gaseous outlet
The least mature technologies have been investigated by e.g. Knuutila, Svendsen and Asif [8] who investigated UV treatment of MEA, DEA and wash water and Knudsen et al [9] who have addressed acid wash and also developed a new concept for reducing mist out of the absorber. The initiatives show that amine emission from gas power based capture in the order of ppb (parts-per-billion) is feasible.
Through the work on measuring amine and amine transformation products, sampling and analytical methods are being developed to be able to both identify and quantify nitrosamines and nitramines in process liquids and in emissions. This includes the construction of a sampling train including isokinetic sampling, sample handling, preservation, storage and transport [10] and the following analytical methods: a total nitrosamine analysis, a screening method for nitrosamines based on chemiluminescence, a quantitative method for analysis of both individual and groups of nitrosamines based on GC-MS [11] and a quantitative method for analysis of 5 nitramines based on LC-MS [12]. As more specific nitramines are being known and synthesized, analytical methods for a larger set of nitramines are expected to evolve. The total nitrosamine [13] analysis has very low detection limit and is suited for quantifying the unknown nitrosamines in the solvent and in emission samples. Online measurements by use of PTR-ToF-MS instruments have been used in a series of projects on atmospheric degradation, see next section, and will now be used at TCM for quantitative measurements of emissions from the CO2 absorber unit as well as wider field measurements [14]. This instrument has limit of detection of 0.1 ppb for amines, nitrosamines and nitramines.
2.2 Atmospheric formation, destruction and dispersion
The first assessment with worst case estimates for Mongstad [1] was based on available literature data. There was e.g. no data for transformation of MEA in the atmosphere and a 2% conversion to nitrosamines was adapted from literature data on triethylamine [1]. The assessment was also based on a simplified modeling approach that e.g. assumed instantaneous conversion of amines at stack exit and no further degradation of harmful components in relation to their environmental fate. The assessment showed that amine emission from capturing 1 million tons of CO2 per year from a combined cycle gas power plant could cause a risk to humans and the environment.
The study showed that there was little experimental data on atmospheric transformation of amines relevant for CO2 capture as well as few data on further degradation and lifetime of the formed harmful components. In 2009 University of Oslo started their Atmospheric Degradation of Amine (ADA) program that included experimental investigations in the European Photo Reactor, EUPHORE in Valencia, Spain. The program (2009 to 2011) has investigated and quantified the potential for forming nitrosamines and nitramines of eight amines: MEA, methyl-, dimetyl-, trimethyl-, ethyl-, diethyl, triethyl-amine and piperazine [15,16]. The various components were identified and quantified by offline analysis and adsorbents as well as by online FT-IR and PTR-ToF-MS measurements. The photolysis of nitrosamines and atmospheric lifetime of nitramines were also part of the program. By use of QSAR (Quantitative structure activity relationship) models this comprehensive set of data provides input for theoretical modeling of other amines relevant for CO2 capture.
Other findings were that primary amines will not form nitrosamines, however, nitramines might form from primary amines both in process and in atmosphere. The potency to form nitrosamines and nitramines has been found to be increasing in the following row for primary < secondary < tertiary amines respectively. Volatile alkylamines can also form from the alkanolamines used in the capture process and be released to atmosphere where further transition to nitrosamines and nitramines could occur [15,16].
Atmospheric transformation, destruction and dispersion has been further investigated and applied through the environmental permit for TCM and as part of technology qualification amine program. The environmental permit for TCM, see Maree et al [17], includes limits for both the emission to air at absorber exit and the calculated yearly average of sum of nitrosamines and nitramines in the nature. These values shall not exceed 0.3 ng/m3 and 4 ng/litre for air and drinking water, respectively. This is according to recommendations from Norwegian Institute of Public Health (NIPH) [18] and based on the toxicity of the nitrosamine NDMA. The concentrations in air and drinking water are obtained by modelling atmospheric chemistry, dispersion, deposition by precipitation and degradation in fresh water with methods that were employed by Norwegian Institute for Air Research (NILU) [19,20]. The risk levels as depicted by the NIPH guidelines for nitrosamines and nitramines decreased as the NILU model developed [21]. The first sets of assumptions to the model were largely conservative in which the chemistry was assumed to happen instantaneously with no degradation of species once they are formed. These set of result were dubbed the “worst case” study”, but with growing knowledge on the chimestry mechanisms as well as degratation of nitrosamine and nitramine spieces a more “likely case” study was developed [19,20]. The latter showed a significant decrease in risk and which showed that the process will conform to NIPH guideline quality criteria as presented in Table 1.
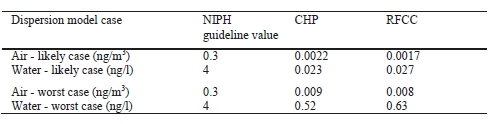
Table 1. Risk level comparison of nitrosamine and nitramine concentrations (sum) with update in dispersion model assumptions at TCM, adapted from [21]. Two flue gas sources: CHP with 3.5 % CO2 from a combined cycle gas power plant and RFCC with 13 % CO2 from the residue fluid catalytic cracker.
The “likely case” model and values, as presented in Table 1, accounted for degratation of nitramines and nitrosamines in both air and fresh water. Results from biodegradation studies conducted by SINTEF [22] on the nitrosamines NDELA, NDMA and nitrosopiperazine (NPz) showed significant biodegradation of these species. Nitramines, on the other hand, exhibited significant lower biodegradability [21].
CCM did during 2011 continue the study on atmospheric chemistry of amines and closed knowledge gaps related to both daytime and nighttime specific amine, nitrosamine and nitramine chemistry as well as chemistry in the aqueous phase in the atmosphere. Table 2 indicates what has been studied and what has been found less important pathway not necessary to pursue when chemistry models were to be put up and introduced in dispersion models.
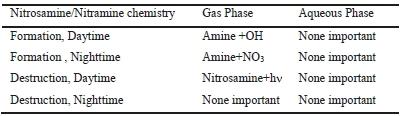
Table 2. Reaction pathways of amines, nitrosamines and nitramines studied.
For the most important reaction pathways all necessary data to decide reaction rates and quantities has been found through experiments and tests with the generic amines [15,16]. In this way reaction schemes with corresponding data can be included in dispersion models to enable simultaneous dispersion and chemical reaction of amines and the corresponding nitrosamines and nitramines in the atmosphere. Table 2 also shows that reactions in the aqueous phase were not found to be of importance neither for the formation or destruction of nitrosamines and nitramines. On the other hand the high solubility of capture type amines will pull the amines out of the gas phase reaction conditions in relation to degradation and they will not undergo reactions in the aqueous phase. As such partitioning of amines to the aqueous phase in the atmosphere will constitute an important loss process for capture related amines [16].
Cambridge Environmental Research Consultants (CERC) and DNV have in parallel developed two different dispersion models, ADMS- an advanced steady state gaussian plume model capable of treating simplified chemistry schemes and Calpuff a modified gaussian puff model with several chemistry schemes included. By introducing chemistry in dispersion models it has been shown that the maximum ambient air concentration of sum nitrosamines/nitramines can be reduced by a factor of 10 relative to the assumption of instant formation at the outlet of the stack. Simultaneous dispersion and chemical transformation is thus considerably slowing down the formation rate of nitrosamines and nitramines [23,24]. At TCM Leibniz Institute for Tropospheric Research (IfT) has been engaged to further develop dispersion modeling by use of the COSMO-MUSCAT model. NILU is currently introducing amine chemistry into WRF-Chem (Weather Research and Forecasting Chemistry) in a CLIMIT project. Thus, several approaches for dispersion modeling with amine chemistry are currently developed in parallel.
2.3 Deposition, exposure and toxicity
Most amines and amino acids used in carbon capture are highly soluble in water. The same is expected for the corresponding nitrosamines and nitramines [25]. It is thus expected that these will dissolve in rain and fog droplets and ultimately be precipitated and end up in surface water, rivers and lakes.
The baseline studies conducted by the Norwegian Institute for Water Research (NIVA) and NILU were not able to show detectable amounts of nitramines or nitrosamines in the environment surrounding TCM at Mongstad. The surveillance activities did, however, detect amines in air, soil, moss and water [26,27]. Table 3 summarises the detection of a selection of amines in air, soil, moss and fresh water. Continuous monitoring in air, aquatic and terrestrial environments surrounding the TCM plant will be conducted on a 2 yearly basis.
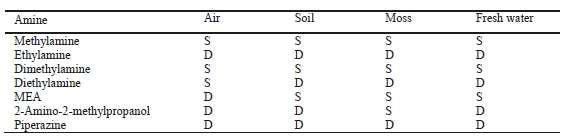
Table 3. Detection of amines in air, soil, moss and fresh water as part of the baseline surveys by NIVA and NILU [26,27]. refers to levels at or below detection limits that are 0.01 ng/m3 for air, 1 ng/g for soil and moss and 10 ng/g for water. significant levels detected.
Literature reviews have revealed that approximately 90% of the about 300 nitrosamines tested have shown carcinogenic effects in bioassays or laboratory animals [18]. A genotoxic mechanism has been confirmed which implies a non-threshold approach in the risk assessment. Existing data on 23 nitrosamines which might form during carbon capture has been reviewed and IARC (International Agency for Research on Cancer) has classified 2 of them as probably carcinogenic to humans and 7 of them as possibly carcinogenic to humans. There is considerably less data on nitramines but they are suspected carcinogenic. None are however classified by IARC. Animal carcinogenicity studies have been carried out for two nitramines: methylnitramine and dimethylnitramine. These results indicate that dimethylnitramine is to be considered at least 6 times less toxic than the corresponding nitrosamine, NDMA.
Due to the very limited amount of toxicity data available on nitramines a toxicity test campaign has been initiated [12]. The following five were investigated: dimethyl-, methyl-, MEA-, AMP- and piperazine-nitramine. Included in the test were acute toxicity, cytotoxicity, skin and eye corrosion, sensitization as well as genotoxicity in three different assays. Overall, the results showed very low toxicity levels. However, three out of the five tested positive on genotoxicity. The next step is to get a more quantitative evaluation of selected nitramines and rank them in relation to nitrosamines. This can give input to a more refined and less conservative toxicity evaluation than the one of today were all nitrosamines and nitramines are added and weighted equally toxic as one of the most toxic nitrosamines (NDMA).
2.4 Framework for qualifying amines for CO2 capture
The knowledge gathered through the above explained studies, methods, models and procedures, will constitute a scientific framework for qualifying amines for use in CO2 capture processes. This framework or toolbox is already in operation through CCM for Mongstad site.
3. Conclusion
The comprehensive investigations reported above have greatly reduced the uncertainty about health and environmental risks resulting from amine-based CO2 capture. Valuable new know-how on amine technology, of high scientific and technical quality, has been generated and new sensitive methods for sampling and analysis of solvent and emissions have been developed. Atmospheric fate of emissions has been studied with regard to dispersion, transformation and degradation, and main knowledge gaps have been closed. The potential toxicity (carcinogenicity) of amine degradation products has been reviewed and tested. Finally, program for amines is established as a valuable toolbox and the program is currently in operation with participation of four vendors.
Acknowledgements
The authors would like to acknowledge all the institutes and researchers that have contributed to the comprehensive set of studies and the institutes and companies that have supported this financially.
References
- Karl M, Brooks S, Wright R, Knudsen S. Amines Worst Case Studies Worst Case Studies on Amine Emissions from CO2 Capture Plants (Task 6). NILU: OR 78/2008.
- Environmental studies by CLIMIT-program: http://www.climit.no/infocenter-2/environmental-studies/
- Environmental studies by TCM: http://www.tcmda.com/en/Technology/Environmental-studies/
- Environmental studies by CCM: http://www.gassnova.no/co2-handtering/miljoeffekter
- Fostås B, Gangstad A, Nenseter B, Pedersen S, Sjøvoll M, Sørensen AL. Effects of NOX in the Flue Gas Degradation of MEA, Energy Procedia, 2011,4: 1566 1573.
- Einbu A, DaSilva E, Haugen G, Grimstveit A, Lauritsen KG, Zahlsen K, Vassbotn T. A new test rig for studies of degradation of CO2 absorption solvents at process conditions; comparison of test rig results and pilot plant data for degradation of MEA. Pending article for GHGT-11, 2012.
- Kolderup H, DaSilva E, Mejdell T, Tobiesen A, Haugen G, Hoff KA, Josefsen K, Strøm T, Furuseth O, Hanssen KF,Wirsching H, Myhrvold T,. Johnsen K. Emission reducing technologies, Sintef report A 18095, 2011.
- Knuutila H, Svendsen HF, Asif N. Destruction of nitrosoamines with UV-light. Pending article for GHGT-11, 2012.
- Knudsen JN, Bade OM, Anheden M, Bjorklund R, Gorset O, Woodhouse S. Novel concept for emission control in post combustion capture. Pending article for GHGT-11, 2012.
- Wittgens B, Enbu A, Brunsvik A, Zahlsen K, Hunnes E, Johansson. Establish sampling and analytical procedures for potentially harmful components post combustion amine based CO2 capture. Sintef report F 16586, 2010.
- Zahlsen K, Brunsvik A, Wittgens B, Einbu A. Literature survey of analytical procedures and recommendations. Sintef report F 16585, 2010.
- Dye C, Fjellsbø LMB, Dusinska M. Nitramine analysis procedure development and screening toxicity study, NILU OR15/2011.
- Ning D, Shah A D, Lanhua H, Plewa M J, McKague B, Mitch W A. Measurement of Nitrosamine and Nitramine Formation from NOX reactions with Amines during Amine-based Carbon Dioxide Capture for Postcombustion Carbon Sequestration, Pending article Environmental Science & Technology, 2012.
- Schade GW, Zhu L, Nielsen CJ. PTR-ToF-MS: Quantitative on-line emissions measurement. University of Oslo report to TCM DA. Technical report 2012.08.14.
- The ADA reports can be downloaded from: http://mn.uio.no/ada
- Nielsen CJ, Herrmann H, Weller C. Atmospheric chemistry and environmental impact of the use of amines in carbon capture and storage (CCS). Chem. Soc. Rev., 2012, 41, 6684-6704.
- Maree Y, Nepstad S, De Koeijer G. Establishment of knowledge base for Emission Regulation for the CO2 Technology Centre Mongstad. Pending article for GHGT-11, 2012.
- Låg M, Lindeman B, Instanes C, Brunborg G, Schwarze P. Health effects of amines and their derivatives associated with CO2 capture. The Norwegian Institute of Public Health, 2011 ISBN 978-82-8082-462-2.
- Berglen TF, Tønnesen D, Dye C, Karl M, Knudsen S, Tarrasón L. CO2 Technology Centre Mongstad updated air dispersion calculations. NILU OR 41/2010.
- Tønnesen, D., Update and Improvement of Dispersion Calcultations for Emissions to Air from TCMs Amine Plant. NILUOR 52/2011.
- De Koeijer G, Talstad VR, Nepstad S, Tønnessen D, Nielsen CJ. Health risk analysis for emissions to air from CO2 Technology Cen tre Mongstad. Pending article, 2012.
- Brakstad OG, Booth A, Zahlsen, K. Biodegradation of nitrosamines in water. 2011 Sintef report A18258, 2011.
- Fowler T, Graham V, Executive Summary, Atmospheric Chemistry Modelling of Components from Post-Combustion Amine-Based CO2 Capture, DNV, Ref.no. PP011013, May, 2012.
- Price C, Atmospheric Chemistry Modelling, Executive Summary, CERC, FM901/ES1/12, May, 2012.
- DaSilva E, Booth A, Loeppky R, Ma`Mun S, Matthias K, Heimstad E, Johnson M, Feilberg K, Protokol for evultation of solvents process and atmospheric chemistry, Sintef report, 2010-06-19.
- Grung M, Ranneklev S, Garmo Ø, Wright RF, Myking T, Heegaard E, Øyen B-H, Schei FH, Blom HH. Terrestrial and aquatic baseline study and monitoring programme for CO2 Technology Centre Mongstad. NIVA 6311-2012.
- Tønnesen D, Dye C, Bøhler T. Baseline study on air and precipitation quality for CO2 Technology Centre Mongstad. NILU OR 73/2011.